7 ways the Webb Telescope will unlock the Solar System's secrets
It wasn't just built for deep space.
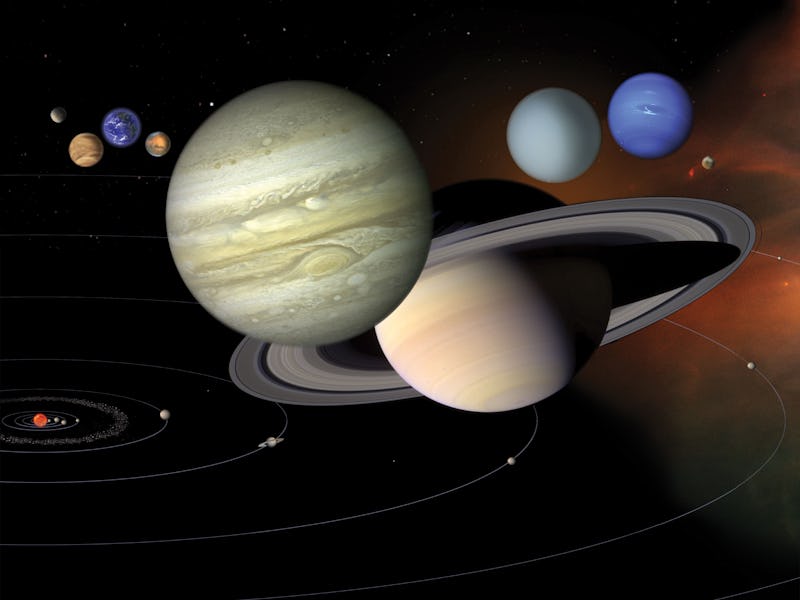
If you know anything about the James Webb Space Telescope, it’s probably that the telescope is designed to view very, very distant things.
NASA’s new flagship science mission possesses instrument sensitivity reaching into the far-infrared, and an enormous, 21-foot diameter primary mirror — tools exceptionally well-tuned for imaging, say, the first galaxies to form in the universe billions of years ago, or characterizing the atmospheres of exoplanets.
But the same tools that can be turned on impossibly distant alien worlds can also be turned on those closer to home — like the composition of relatively nearby alien worlds, perhaps even a nearby ice giant that’s only been visited once by human spacecraft (Voyager 2, in 1986).
“It's like [Webb] was designed to study Uranus’s atmosphere — it's fabulous,” Heidi Hammel, an interdisciplinary scientist on the James Webb Space Telescope project, tells Inverse. “I used to tease my extra-galactic colleagues, ‘thank you so much for building this camera designed to do Uranus work!’”
It’s not just Uranus. Around 7 percent of the observing time in Webb’s first year is dedicated to Solar System science, with 22 proposals from the planets science community to train Webb on everything from asteroids to the Jovian cloud tops. And as an interdisciplinary scientist involved with Webb since the early 2000s, Hammel has 100 hours of guaranteed observing time to herself, and she’s going to make a grand tour.
“It's basically a sampler of everything in the Solar System,” she says.
Resources are limited, and when it comes to visiting all the places in our Solar System planetary scientists would like to study, they might as well be billions of light years away. So it’s fitting Webb will help advance astronomy in our own backyard at the same time it takes astronomers back to the distant infancy of the universe.
What Webb can see in the Solar System?
Webb will orbit the Sun in a stable position relative to our planet, stationed in an orbit around Lagrangian Point 2, a position about 1 million miles from Earth. This deep-space perch is essential to Webb’s operations, as heat from the Sun or Earth can blind its exquisitely sensitive infrared instruments. Webb will keep its back to us and our star permanently, protected by a tennis court-sized sunshield.
Consequently, Webb can’t take images of the Sun, Mercury, Venus, Earth, or the Moon. But anything else is fair game, including near-Earth asteroids and the rest of the planets, beginning with the red one.
Given its larger mirror, Webb will be able to glean more about Mars than Hubble, which took this photo.
7. How Webb will observe Mars
“People say, ‘why would you look at Mars? Haven't we sent eleventy-thousand spacecraft there?’” Hammel says. “Yes, we have. But Hubble has actually been pretty powerful looking at Mars, and Webb will too.”
Given its larger primary mirror, Webb will have greater spatial resolution than the Hubble Space Telescope when viewing Mars, but Hammel points out that Hubble was a largely visible spectrum instrument. Where Webb really shines is in the infrared, particularly infrared spectroscopy, which can allow scientists to break down the chemical composition of distant objects.
“We will be able to map out surface properties of from Mars, both during the daytime and the nighttime,” Hammel says, mapping out dust and water clouds, but also organics such as methane. Methane levels seem to vary from measurement to measurement on Mars, she says, and Webb spectroscopy should ascertain whether it’s varying across the entire planet with time, or if methane varies across regions on the Red Planet.
6. Webb and the asteroids
If you look at some of the Hubble Frontier Images where the venerable space telescope attempted to image distant galaxies, you’ll see weird arcs across the picture, like strange scratches on a physical photograph keep too long in a junk drawer and subjected to thrown keys and coins.
“All of those weird arcs are asteroids that have photobombed this picture of galaxies,” Hammel says. But the unwanted noise for Hubble will be data for Hammel and other Solar System scientists with Webb, “And although we will not resolve pictures of asteroids, we will be able to get spectroscopy of the asteroids,” she says.
Asteroids interest planetary scientists as they hold information about the earlier days of our Solar System, offering looks at the same sorts of rocky materials that once agglutinated to form Earth and the other rocky planets. Scientists want to understand the composition of asteroids, and have studied them using ground based-spectrographs.
There’s a catch though: the spectra of most asteroids look fairly similar, except for a region of the spectra that “is completely obscured from the ground,” Hammel says. This is a problem for a lot of Solar System spectroscopy — the spectral bands of interest for some distant object align with the spectra of something in Earth’s atmosphere, like water vapor, which knocks gaps in the spectra of your distant object.
“There are only certain windows of light that get through to the ground,” Hammel says. “If you want to see stuff inside those blocked windows, you have to go to space.”
Webb to the rescue. Once again a design suited to viewing distant galaxies will serve exceptionally well closer to home, allowing astronomers to differentiate asteroids like never before, using complete spectra to pinpoint the temperature and pressure at the time each asteroid formed.
Jupiter and Europa as seen through Hubble.
5. Webb’s tricky view of Jupiter and Saturn
Space-based infrared spectroscopy will be a boon when Hammel and her team turn their attention to the giant planets as well. While Webb will help scientists better understand certain structural features of Jupiter and Saturn’s atmospheres, Hammel is most interested in better understanding the chemistry, particularly color chemistry, of the showy gas giants.
“We're going to be looking at the Great Red Spot and seeing if we can figure out what the chromophore is what gives it that red color,” Hamel says.
But Jupiter and Saturn are large, relatively close to our Sun, and therefore very bright. It’s going to take some technical workarounds, Hammel says, for Webb to even take a peek at them.
“Remember, these detectors are designed to sense the faintest galaxies in the universe,” she says. “We have to use special tricks to look at very bright objects, like special wavelengths that we know are not going to saturate the detectors.”
4. Webb and icy moons
NASA’s Europa Clipper mission is scheduled to launch sometime in 2024, arriving at Jupiter’s moon Europa to do spectroscopy sometime in 2030.
But why wait? Hammel wants to use Webb’s spectrometer to try and characterize the composition of what are believed to be jets of water erupting from Europa, performing the same observations for similar plumes of water detected escaping the south pole of Saturn’s moon Enceladus by the Cassini mission. Both Moon’s are believed to harbor subsurface global oceans in contact with rocks and minerals and warmed by geologic activity — ingredients that could make up a recipe for alien aquatic life.
“We're going to try to put our beam, our detector on the area where those plumes should be, and try to do spectroscopy,” Hammel says. “It's risky. Not risky, dangerous to the spacecraft. Risky in that we may not detect anything.”
But that, she says, is the beauty of being an interdisciplinary scientist with guaranteed time.
“Potentially risky observations may not make it through a traditional time allocation committee,” Hammel adds, but “this is why I’m doing it — I have guaranteed time and no one can tell me no. That’s the beauty of guaranteed time and part of the reason they give it to us.”
Webb will also allow Hammel and other scientists to pick up on other threads of Saturnian science left by the Cassini mission, particularly explorations of Saturn’s largest moon, Titan.
“It's enshrouded in a photochemical smog,” Hammel says. “It's a thick atmosphere, but the beauty of an infrared wavelength is infrared wavelengths can penetrate through that smog and give you surface detail.”
Webb will allow scientists to map the existing dunes and hydrocarbon lakes on Titan through the smog, and then watch them change over time.
3. Webb and the Ice Giants
One of the reasons Hammel jokes that Webb was tailor-made for studying Uranus is that the four different fields of view of Webb’s Mid-Infrared Instrument (MIRI), each corresponding to different infrared wavelengths, perfectly frame up the side-tilted ice giant. This will help Hammel and other planetary scientists better study Uranus’s upper atmosphere and understand how it interacts with the lower levels they can better image with existing telescopes.
And of course, spectroscopy will allow for the study of Uranus’s chemistry, particularly the presence of hydrocarbons.
“This is like a mother lode for us to be able to not only detect these hydrocarbons,” Hammel says, “but to be able to map them out on the surface and figure out like what's driving them and where they come from, in the atmosphere.”
Neptune is a similar target, with Webb enabling scientists to maps its atmospheric structure and how winds, temperature, and clouds correlate with the planet’s chemistry.
Pluto has only been visited once, but Webb could help keep track of seasonal changes on the dwarf planet.
2. Webb and the Kuiper Belt
Once you get out to the frosty, outermost regions of the Solar System, using Webb for local planetary science makes more intuitive sense. Dwarf planets such as Pluto, Eris, and Makemake are very far away, very cold, and scientists very much want to understand what they are made of.
Dwarf planets such as Pluto, Eris, Makemake, and other Kuiper belt objects, are so small, cold, and far away, that they've been left relatively unchanged since the birth of the Solar system, Noemi Pinilla-Alonso, a planetary scientist with the Florida Space Institute tells Inverse. Like asteroids, Kuiper belt objects hold a record of the early Solar System, but in their case, a slice of the protoplanetary disk much further away from the Sun than the material that formed the rocky inner planets.
Pinilla-Alonso is leading a program to closely examine 59 “trans-Neptunian” objects over 100 hours, a full survey designed to understand the range of differences in these distant bodies, and feeding that information into models for the formation and evolution of the Solar System.
“What we are going after is to really have a good representation of the diversity in the trans-Neptunian belt,” she says, “to be able to answer questions that are related to all the population of icy bodies in the solar system, not only to some specific objects.”
1. Webb and comets
Comets split the difference between trans-Neptunian objects and asteroids, falling Sunward from the icy reaches and passing through the inner solar system before passing out again on their long, elliptical orbits.
They also represent targets of opportunity for Webb, which can, once again, use spectroscopy to tease apart their structure and chemical composition.
“Whatever comet is up, we have a program that is designed to track comets in general; we call them ‘target of opportunity programs,’” she says.
And not just comets.
“If another interstellar object comes by like Oumuamua, there's already a program approved to do that work as well,” Hammel says. “If we get another impact on Jupiter, we're ready.”
When will Webb start looking around the Solar System?
Webb has another five to six months of calibrations and commissioning tasks before it’s ready to do science, so for the time being, Hammel has team members looking to see what Solar System objects might be visible for Webb around the time it comes online and her observations can begin. If they have a clear line on Jupiter, they might star there, or they may start with Mars.
“Kuiper Belt objects are everywhere, so my guess is that we'll have some Kuiper Belt objects, probably within the first two months of when we start doing observations,” Hammel says. “It just depends on where things are in the sky.”