Muon g-2 experiment results: Physicists may have discovered a new force of nature
The answer may lay beyond physics as we know it.
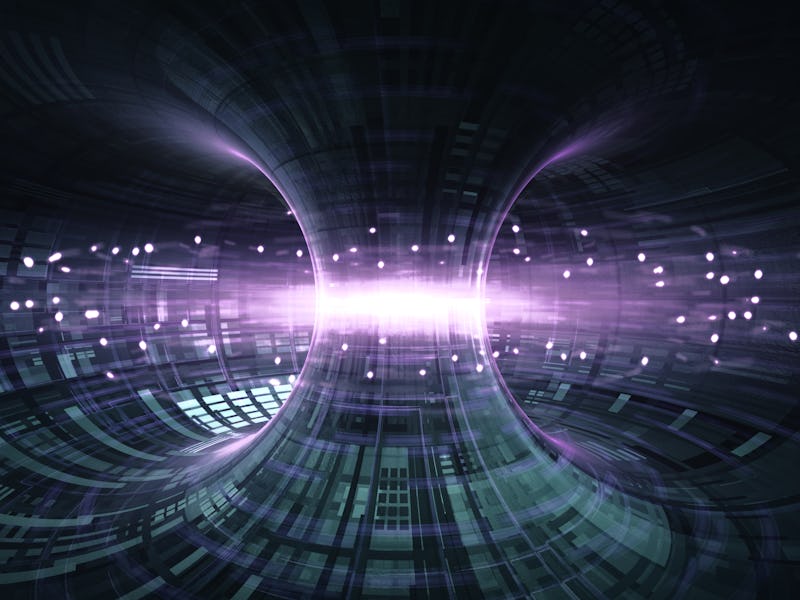
In the world of particle physics, you are never alone — quite literally.
Every moment there is an invisible rainstorm of subatomic particles falling down on us from space. Unlike the kind of matter we’re used to — which puts up a fight if pushed up against — these tiny particles can sail right through us and straight into the Earth’s core.
As far as physicists know, these particles aren’t causing us any harm or even giving us a tickle as they pass through unnoticed like ghosts. But the strangeness of these particles may hold a secret key to answer the universe’s most persistent questions, like the origin and essence of dark matter.
Suspect number one at the center of the particle-filled mystery? The humble muon.
What is a muon?
A muon is a type of high-energy particle called a lepton. Unlike particles like protons which are made up of electrons and quarks, leptons are considered “fundamental particles,” meaning they’re not composed of any smaller parts.
Like the magnets you might find stuck to a fridge, these tiny particles all have their own magnetic field and spin. The strength of this personalized magnetic field is referred to as the particles’ “magnetic moment” or its “g-factor.”
Like fridge magnets, high-energy particles generate their own magnetic fields called their “magnetic moment” or “g-factor.”
In addition to muons, the lepton group also includes the particle tau and the common electron. In fact, muons in many ways are the heavier and stranger cousin of the electron. Even though electrons and muons have the same negative charge, a muon is more than 200 times heavier.
Muons are also much more ephemeral than their electron counterparts. While scientists have found electrons to be essentially immortal, muons only exist for 2.2 seconds before decaying (a scientific term for breaking down into pieces) into electrons and neutrinos.
While they live much shorter lives than electrons, the lifespan of the muon far exceeds that of other leptons like tau. This makes the muon a kind of Goldilocks particle that particle physics can use as a proxy to study the essential nature of other high-energy particles.
“It... potentially points to a future with new laws of physics.”
Experimenting on the muon — Since their discovery in the 1930s, scientists have done a number of experiments to capture and study this elusive particle. One of the most notable theories and experimental predictions about the muon was made by British physicist Paul Dirac.
According to the self-named Dirac Equation — which describes the motion and electromagnetism of particles using both quantum mechanics (the action of the smallest particles in the universe) and special relativity (the relationship between space and time) — the magnetic moment of a muon is exactly equal to two. This was confirmed by experiments at the time.
Crucially, the Dirac Equation appeared to accurately predict not only muons’ spin but that of all other particles as well — a metaphorical home run for theorists.
But little did scientists of the 1930s know that the journey to understand the muon was only just beginning. Starting in the 1940s and 50s and continuing to today, scientists have observed extra-magnetism being emitted from the muon that cannot be explained by Dirac’s equation — or any other equation to date.
This open question is called the muon anomalous magnetic moment.
How is the muon anomalous magnetic moment studied?
Scientists at CERN began studying the magnetism of muons in the 1950s, but in the 1990s this research moved stateside, first to Brookhaven National Laboratory in Long Island until 2001 and now to Fermilab, just outside Chicago. This modern experiment is called muon g-2 (pronounced muon g minus two.)
Shipped from Brookhaven to Fermilab in 2013, the apparatus at the center of this experiment is a superconducting storage ring with a 50-foot-diameter. According to Fermilab, this ring operates at negative 450 degrees Fahrenheit — just several degrees shy of absolute zero — and works by spinning a beam of muons around in circles and detecting where their decay is flung off.
After its stint at Brookhaven National Laboratory, the muon g-2 experiment was moved to Fermilab in 2013.
2021 Muon g-2 results
After nearly twenty years of silence from the g-2 experiment, Fermilab released some of the experiment's newest results this April.
Just like the 1950s researchers at CERN and their Brookhaven colleagues, the initial results of the new Fermilab experiments also found a strange, unexplained excess of magnetism around their muon samples.
“Today is an extraordinary day, long awaited not only by us but by the whole international physics community,” Graziano Venanzoni, co-spokesperson of the Muon g-2 experiment and physicist at the Italian National Institute for Nuclear Physics, said in an April statement.
Does muon g-2 2021 fit the Standard Model of physics?
What excites scientists most about these findings is that it may be just the fodder they need to truly begin to break down the academic monopoly that is the Standard Model (SM) of physics.
First developed in the 1970s, the SM describes the relationship between three out of four of the universe’s observed forces:
- electromagnetism, which governs electrical currents and magnetic fields
- the strong force, which is responsible for subatomic particles like the proton and the neutron staying glued together from their component parts
- the weak force, which causes particles to decay
Predictions made using the SM fits most observable scenarios, with one glaring omission: it doesn’t jive with the gravitational force, which is much weaker than the other forces. The SM also fails to explain the abundance of dark matter and dark energy observational found in the universe.
These muon discrepancies may provide scientists enough ammo to push beyond the limitations of the SM.
Can the muon magnetic moment experiment reveal new physics?
One such new pathway opened by this deviation from the SM would be a better understanding of what scientists call a “quantum foam” which may be driving the muons’ strange magnetism. Essentially, scientists theorize that this foam consists of “virtual” particles that pop quickly in and out of existence around better-understood particles like muons.
Transported halfway across the country, Fermilab now uses this superconducting magnetic storage ring to study the magnetism of muons.
Any interactions with either unknown forces or particles within this quantum foam could be responsible for throwing muons’ g-factor off kilter. Such forces or particles wouldn’t currently fit within the SM.
How is the muon anomalous magnetic moment connected to a fifth force of nature?
Scientists at Fermilab are still only just beginning to analyze the swath of data they’ve collected from this new muon g-2 experiment, but if the quantum foam is truly the root cause of the muon anomaly then physicists are beginning to speculate that this foam may indeed hide a mind-bending scientific discovery.
“This is very exciting because it potentially points to a future with new laws of physics, new particles and a new force which we have not seen to date,” Mark Lancaster, the UK lead for the experiment, told BBC News in April.
This article was originally published on