Jupiter's core hides a disturbing cannibal past — study
New evidence shows Jupiter ingesting large quantities of planetesimals.
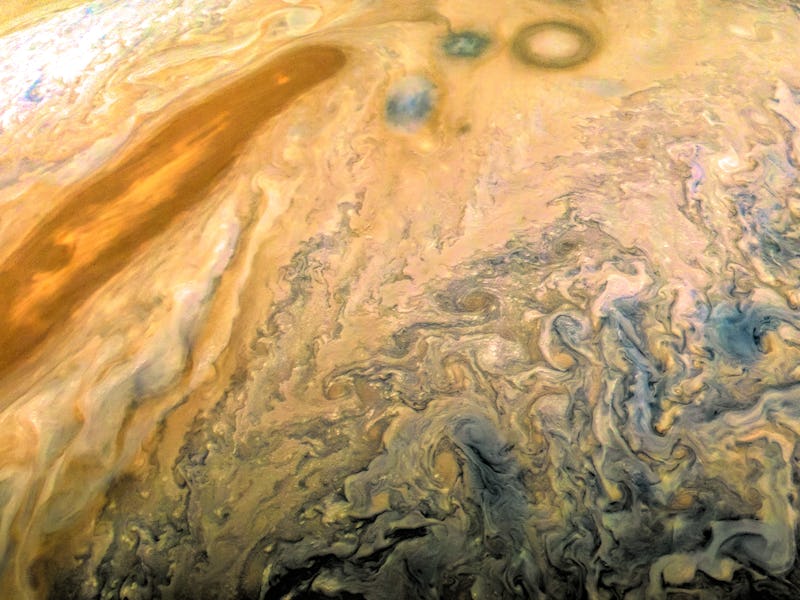
More than a decade after its launch, observations from NASA’s Juno probe — which embarked on a four-year extended mission last year — have revealed that the interior of Jupiter is far different than scientists had previously thought possible. The possible reason? The Solar System’s largest planet was formed by devouring planetesimals, the smallest baby planets.
While Juno is far from the first probe to visit Jupiter’s system — Pioneer 10 was the first of the gas giant’s many flyby visitors nearly 50 years ago, and Galileo spent seven years orbiting the planet — it is the first to be able to make the kind of measurements of the world of extreme pressures and temperatures far below the cloud tops that reveal the earliest days of the biggest planet.
A new paper from an international team of astronomers led by Yamila Miguel at the Leiden Observatory in the Netherlands studying Jupiter’s core published in Astronomy and Astrophysics sheds light on the giant’s birth. Data from Juno’s instruments has allowed them to model the planet’s deepest core, revealing the planet’s beginnings in the process–and raising new questions not just about the makeup of all the gas giants but about the basic physics of the extreme environments at their cores.
Here’s the background — Historically, astronomers assumed that Jupiter’s interior was one of two things once you got below its outermost layers:
- A relatively small core, still many times the mass of Earth, made of metals (what astronomers call any element heavier than helium) surrounded by an atmosphere of helium and hydrogen,
- A blend of elements mixed well by the rise and fall of convection all the way through.
Newer data from Juno suggests that Jupiter’s core is surrounded by distinct inner and outer atmospheres divided at the point where the helium separates from the hydrogen and falls as rain.
Some of the previous hypotheses around Jupiter’s interior, which Juno has complicated.
What’s new — Yamila Miguel, who spearheaded the paper, tells Inverse that measurements from Juno’s gravitational instruments indicate that the inner atmosphere is not just hydrogen and helium, but is filled with heavy metals that did not fit any previously created model — or, for that matter, the equation of state, the thermodynamic equation describing the way hydrogen behaves under extreme pressures and temperatures. This inner atmosphere is much heavier than anyone had anticipated, between 11 and 30 times the total mass of Earth.
These measurements indicate that Jupiter must have been formed from the miles-long building blocks of planets in the early Solar System called planetesimals.
A competing model astronomers use is a sort of accumulation theory of smaller building blocks. These are referred to as “pebbles,” which are actually about the size of boulders. Had Jupiter been formed just through the accretion of tiny pebbles, scientists would expect to find metals mixed throughout the atmosphere. But because the metals are spread in a gradient through a larger dilute core instead, the giant must have continued to devour metal-rich planetesimals instead.
Juno principal investigator Scott Bolton tells Inverse that icy planetesimals, rich in water and heavy elements, would have provided the mixture of elements detected in the planet’s innermost layers. Understanding how Jupiter came to be seeded with heavy elements will also help us to understand how the rest of the planets came into existence.
Because Jupiter was likely the first planet to form, getting a better view of the way it came together is “very valuable information to understand the very first stages of the formation of the Solar System,” Miguel says. Of course, we have no way of observing Jupiter billions of years ago, but understanding the way that Jupiter was formed can help explain if this is the way that the other gas giants formed or if Jupiter is anomalous.
“It was a surprise,” Bolton says, and now the challenge is to understand “How do you make a planet like that? How do you maintain it like that? Or did Jupiter evolve to that?”
These aurora images from Jupiter’s south pole are caused by disturbances deep below.
Why it matters — The measurements Juno has taken of Jupiter’s core reflect the kinds of pressures and temperatures that could only come close to being recreated on Earth if you were studying fusion. And because Jupiter’s core is shrouded by tens of thousands of miles of clouds, gasses, and liquid hydrogen, “the deeper deep interior is very difficult to see with the data we have, and that’s why it’s so difficult to know what happens in the core itself,” Miguel says. What physicists have to do instead is interpret the makeup of the core from indirect observations. But the equations that describe the behavior of the hydrogen, helium, and metals that make up that core are still ambiguous.
The conditions observed by Juno are “very difficult to reach in a lab,” Miguel notes, and because of this our knowledge of what might be going on in there could only come from calculations based on temperatures and pressures that can be created on Earth.
Bolton added that Juno was planned with this in mind, and that the team has long expected that “Juno would be ahead of our fundamental understanding of physics by getting data at Jupiter that would be limited in its interpretation by our understanding of how hydrogen behaves at the middle of a planet.” Miguel adds that data Juno has begun to shrink the ambiguities that researchers faced. “Before, the error bars were so big that the models, you could fit many things in there. But now when we shrink those error bars it means your possibilities to explain the data are way better than before.”
Bolton adds that the central lesson from Juno has been that the understanding scientists get from being up close and personal is very different from the one that they get when observing from afar. When you’re able to get as close as Juno has, he notes, “you learn that the planet wasn’t at all like what you thought.”
This new research shows some of the limits that are going to constrain the study of exoplanets even once the James Webb Space Telescope begins to produce its first images this month: what a gas giant in another solar system looks like on the outside might not be as close to its real makeup as astronomers previously thought.
What’s next — Both Miguel and Bolton emphasize that this work raises more questions than it answers for the time being. These results emphasize the importance of understanding the physics of hydrogen under extreme conditions that cannot be duplicated on Earth.
Bolton notes that Miguel’s work shows what the future study of planets will look like: “not just focused on planetary science as it’s been understood up until now, but unraveling the ways that basic physics and planetary science impact each other,” he says.Before now, it was not possible to take precise enough measurements to show the ways that the planet’s gravity, magnetic field, and deep atmosphere are interacting with each other.
Bolton also noted that these sorts of results will spur similar studies of Saturn, Neptune, and Uranus to understand if the results Dr. Miguel and her team have come up with are unique to Jupiter or if they apply to gas giants more broadly. While the Cassini probe orbiting Saturn does not have the recent instrumentation Juno carries, it has been put into a similar orbit and shows some indications of having a similarly dilute core.
It may be a long wait before researchers have access to this kind of data, however. In the recent Planetary Science Decadal Surve — the survey of scientists NASA uses to help pick future missions — a mission to Uranus was chosen as the most important flagship mission that could be launched in the next ten years.
The white paper proposing the mission takes up the questions raised by Dr. Miguel’s work, including circulation of the ice giant’s atmosphere, the makeup of its core, and more complex 3D probing of its atmosphere. But even if the mission goes ahead, it will be a long voyage to the outer solar system. In the fastest scenario, work on the Uranus Orbiter and Probe would begin in two years in order to launch in 2031 — and it wouldn’t arrive in orbit around Uranus until 2044.
But for now, the models of what the earliest days of gas giants looked like – and what it’s like deep inside their interior — are going to have to evolve. “That’s the fun part,” Bolton says. “It’s a little humbling and sometimes frustrating for some scientists, but it’s also exciting, because that’s partially why we explore.”