Quantum entanglement could fight a disease that kills 600,000 in the US a year
Using "microwave quantum illumination" researchers rely on entangled photons to detect objects.
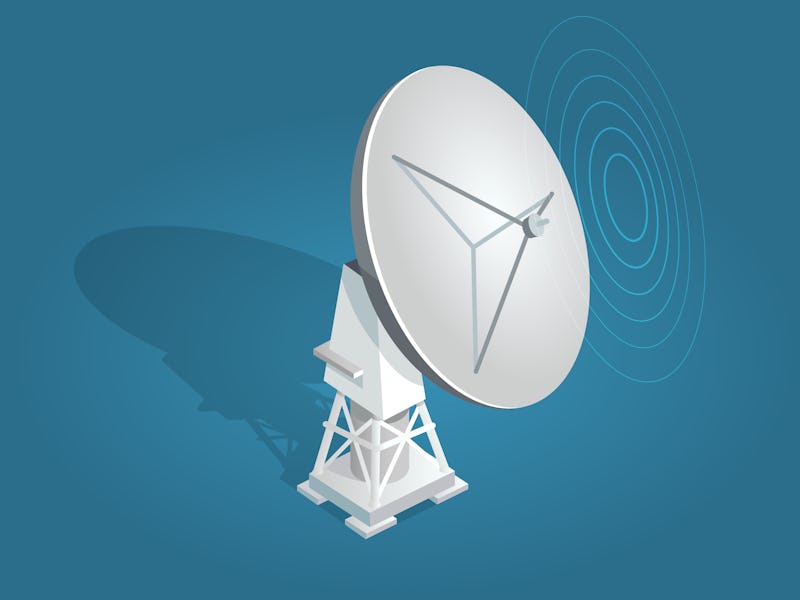
Whether peering back to the beginning of our universe, winning wars, or looking deep inside the human body at dangerous tumors, radar technology has been a reliable extension of human vision for decades. And at 80-years old, thanks to quantum physics, it's about to get even more precise.
Using a supercooled refrigerator and entangled quantum photons, a team of international researchers has designed a proof-of-concept radar that would be more sensitive and less invasive than today's typical models, especially in "noisy" thermal environments. The process is called "microwave quantum illumination."
The researchers say that this could spell big improvements down the road for ultra-low power and sensitive scanners, especially those used for human health. While radar may not be as associated with medical uses as stethoscopes, the technology is used to monitor conditions associated with some of the most deadly diseases in the U.S., including heartbeats, detect strokes, and even identify cancerous tumors. Helping to identify tumor growth alone could improve the lives of nearly 600,000 patients who die each year in the U.S. from cancer.
A study published Friday in the journal Science Advances describes how researchers were able to achieve this sensitivity by taking advantage of a famous property of quantum particles: entanglement.
"Quantum radar or quantum illumination uses a source of entangled microwave radiation, while classical radar uses coherent signals or classically correlated noise signals," lead researcher and professor at the Institute of Science and Technology Austria, Johannes Fink, tells Inverse.
Kind of like best friends with two separate halves of a friendship necklace, entangled quantum pairs are connected to each other even at great distances through residual physical attributes and interactions. That means that information detected by one part of the pair can affect the other, even if they are physically far apart -- something Einstein (fearfully) called "spooky action at a distance."
This quantum radar relies on the relationship between entangled quantum photons to detect objects.
For Fink's team, this spooky action was paramount. Fink tells Inverse that they used a supercooled dilution refrigerator (kept at just over -450 degrees Fahrenheit) to generate a fickle pair of photons, one of which they called the "signal" photon and the other the "idler" photon. In order to take a "look" at any given object, the signal photon was sent out to scout and the idler photon lingered at its origin. Fink tells Inverse that by comparing the information of these two different photons in the pair they could gather precise information about objects in the radar's field.
"One part of the entangled signal is sent to probe an object and the other part of it is kept and stored. A small amount is reflected by the object and this part is also subject to environmental noise," says Fink. "The noisy signal can then be measured together with the stored part of the source signal. Because of the similarity of the two signals (due to the common origin and the strong quantum correlations) a more efficient detection is possible."
Even though the relationship between these photons isn't long-lasting, their joint information creates a signature or pattern of the object that cuts through any thermal noise that may be around it.
"Compared to classical correlated noise, quantum physics allows for stronger correlations to exist at the source, which helps by a certain amount even though the true entanglement is lost before the two signals are recombined (due to the noise)," says Fink.
But that said, while their study has demonstrated the possibility of creating a quantum radar like this, Fink tells Inverse that it's far from fool-proof yet.
In general, Fink says that quantum radars are primarily effective in noisy, close-range environments. Trying to achieve the same accuracy at a longer range wouldn't be possible due to the quantum nature of the photons. Additionally, Fink says that their system right now works best only when very careful calibration is applied to the system, making moving this technique out of the lab more challenging.
"This means we could show a proof of principle working prototype in the lab, but in practice - and without the applied calibration procedure - we would not have been able to outperform the theoretically strongest classical detection strategy," says Fink.
As these challenges continue to be met in years to come, Fink says he foresees two main ways this technology could be used in practice.
"We believe its usefulness is confined to short and medium range applications where the object is not supposed to know (security applications) or notice (sensitive objects like biological tissue) that it is being detected," says Fink.
Abstract: Quantum illumination is a powerful sensing technique that employs entangled signal-idler photon pairs to boost the detection efficiency of low-reflectivity objects in environments with bright thermal noise. The promised advantage over classical strategies is particularly evident at low signal powers, a feature which could make the protocol an ideal prototype for non-invasive biomedical scanning or low-power short-range radar. In this work we experimentally investigate the concept of quantum illumination at microwave frequencies. We generate entangled fields using a Josephson parametric converter to illuminate a room-temperature object at a distance of 1 meter in a free-space detection setup. We implement a digital phase conjugate receiver based on linear quadrature measurements that outperforms a symmetric classical noise radar in the same conditions despite the entanglement-breaking signal path. Starting from experimental data, we also simulate the case of perfect idler photon number detection, which results in a quantum advantage compared to the relative classical benchmark. Our results highlight the opportunities and challenges on the way towards a first room-temperature application of microwave quantum circuits.