What makes your heart tick? Quantum physicists investigate.
Apparently the way to someone's heart is through quantum sensors.
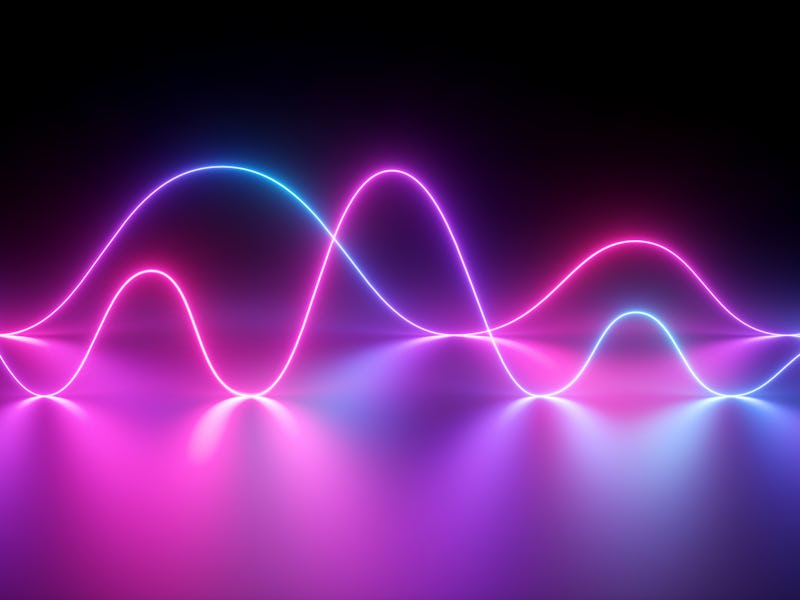
There are many ways to know somebody's heart: long walks on the beach, intimate dinner dates, or incredibly sensitive quantum sensors designed to map their heart's conductivity.
While the latter may not be the best way to get a second date, a team of physicists and astronomers from the University College of London (UCL) say in a new study that it could help cardiologists better identify and treat a common, yet debilitating, heart condition called atrial fibrillation (AF.)
AF affects millions of people in the U.S. every year by causing an irregular or skipping heartbeat that can lead to blood clots, stroke or even heart failure if left untreated. The causes of the condition itself are unknown and clinicians have struggled to develop preemptive and noninvasive ways to treat it. But that could be changing. The authors of a new study published Tuesday in the journal Applied Physics Letters are hoping that borrowing an idea for the world of physics and astronomy may help clinicians better understand this condition.
"[This research] started as a side project, in the main context of security-related or potentially security-related applications, to investigate the feasibility of non-invasive imaging," Luca Marmugi, a co-author of the study and post-doctoral researcher at UCL, tells Inverse. "Then what happened is clinicians from [the UCL] cardiology department approached [us] saying 'We heard that you guys are looking into the possibility of creating conductivity maps for objects -- can you do this with a heart?'."
Marmugi tells Inverse that this approach works by using two different magnetic fields to map the conductivity of an object. In the case of AF, the team would use the sensors to detect any divergence in the initial conductivity map. These points of divergence could then be used by clinicians to pinpoint where a patients' heart was short-circuiting. Marmugi tells Inverse that the super-sensitivity of these sensors is all down to their quantum properties.
"The ‘quantum’ nature of the imaging platform lies in the atomic magnetometer, which the core sensor we use for detecting the very small response field obtained from an object we want to image," says Marmugi. "These sensors are based on laser manipulation and interrogation of atomic spins of Rb atoms... [we] measure the secondary magnetic field produced by an object in response to a small oscillating magnetic field. In this way, we can reconstruct images (or maps) of the electric conductivity. In this case, the ‘quantum-ness’ of the sensor allowed us to overcome the limitations in terms of sensitivity and tunability of conventional sensors."
Authors write that this approach demonstrated 50 percent better imaging quality than previous approaches, and all while operating without magnetic shielding and at room temperature -- a coveted Goldilocks zone in the world of atomic physics.
See also: Honeywell has built the most powerful supercomputer in the world
Marmugi tells Inverse that this technique could also be beneficial in several other medical applications that also use changes in conductivity as a symptom, such a tumor analysis or dermatology.
While the trials described in this study were not yet done on biological tissue, Marmugi tells Inverse that an equivalent synthetic material was used to demonstrate how this technique would be effective in practice. As the technology moves forward toward pre-clinical and clinical trials in the coming months and years, Marmugi tells Inverse that they will continue to escalate their model toward biological tissue, starting with adding more realistic variables like movement from breathing.
"Hopefully, this will lead to the development of the tools that healthcare professionals need for improving the management of such a condition," Marmugi tells Inverse. "In this sense, in the longer term, we hope that this will benefit both ends of the healthcare systems, doctors and patients, with also advantages related to costs containment."
Abstract: Progress in electromagnetic induction imaging with atomic magnetometers has brought its domain to the edge of the regime useful for biomedical imaging. However, a demonstration of imaging below the required 1 Sm1 level is still missing. In this Letter, we use an 87Rb radio-frequency atomic magnetometer operating near room temperature in an unshielded environment to image calibrated solutions mimicking the electric conductivity of live tissues. By combining the recently introduced near-resonant imaging technique with a dual radio-frequency coil excitation scheme, we image 5mL of solutions down to 0:9 Sm1. We measure a signal-to-noise ratio of 2.7 at 2MHz for 0:9 Sm1, increased up to 7.2 with offline averaging. Our work is an improvement of 50 times on previous imaging results, and demonstrates the sensitivity and stability in unshielded environments required for imaging biological tissues, in particular for the human heart.