Quantum gravity sensors could finally overcome a major issue raised by Einstein
Cartography could be changing forever as an advanced tool moves from the lab to the real world.
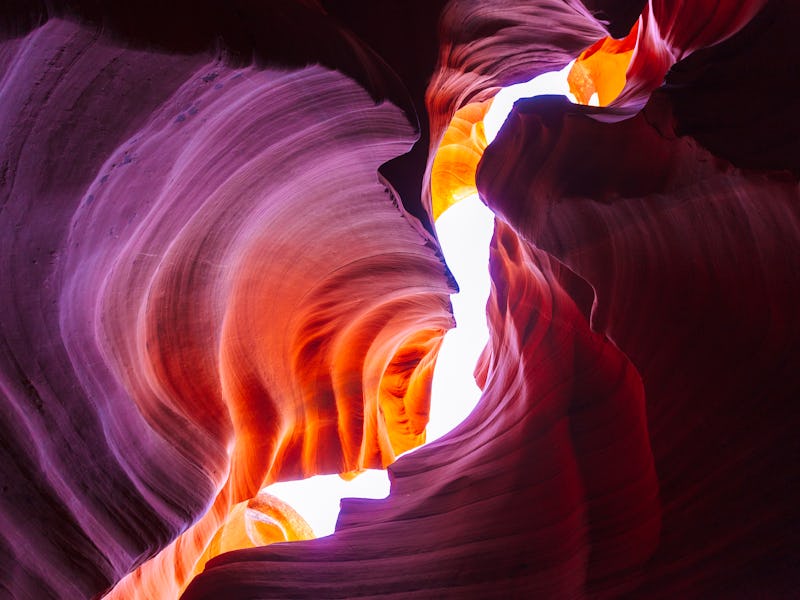
When it comes to understanding the fundamental forces of the universe, gravity is by far the most intuitive. It’s the force that brings us splashing down after jumping from a diving board and the force that sends the Earth on its slow journey around the sun. In recent years, sensing gravity in the form of gravitational waves has also helped scientists peer deep into the universe.
Now, physicists and civil engineers are advancing another way to use gravity in the form of a quantum gravimeter designed to read gravitational fields to “look” deep underground without ever picking up a shovel. In a paper published Wednesday in the journal Nature, Mike Holynski, head of atom interferometry at the University of Birmingham, and colleagues present the first quantum, or atomic, gravity gradiometer capable of taking measurements outside the controlled environment of a mechanics lab.
“Gravity is one of the best ways of looking into the ground, particularly when wanting to look deeper than the first few meters,” Holynski tells Inverse. “This presents a route to gravity sensors which can much more rapidly scan areas, enabling gravity cartography at high resolution, which could benefit applications such as infrastructure monitoring, water resource monitoring, and climate sciences.”
—
Here’s the background — Using gravity sensors to peer under the Earth’s surface is not a new practice; in fact, it’s been going on for over 20 years. In a nutshell, these devices work using interferometry (similar to that used to detect gravity waves at LIGO) to compare the paths that atoms take when a laser pulse sends them on two different paths. Comparing the journies of these atoms can then help scientists understand how local differences in gravitational fields impacted their path.
“In particular, [the sensor] is sensitive to differences created by local differences in density or mass, such as pipes or tunnels under the ground, which create a slightly different gravitational pull for the atom as it travels along the trajectories,” Holynski explains. “[This] allows us to detect things under the ground when we move the sensor.”
In other words, atoms that travel through dense dirt will bring back different gravity signals than those that sailed through an open, underground tunnel. Creating a gravity map from this data can help researchers “see” underground.
However, the sensitivity of these sensors has traditionally been problematic because the vibration noise of a nearby car can be indistinguishable from gravitational changes. Holynski says this is thanks to Einstein’s equivalence principle, which says it's not possible to differentiate between acceleration and gravity.
Albert Einstein’s equivalence principle means traditional gravity sensors can produce misleading results.
That’s where Holynski and colleagues' updated sensor comes into play. Instead of performing this gravity measurement on a handful of atoms, the team’s quantum gravimeter performs these measurements on thousands of atoms that are held in two matching atomic “clouds” within their sensor.
“The vibration measured by each cloud is identical,” Holynski says. “However, because they are a different distance away from a given mass or lack of mass (such as the tunnel in this paper), the pull of gravity is different for the two clouds. We then subtract the two readings – this removes the unwanted noise due to vibration, but maintains useful gravity information.”
Why it matters — Improving the noise reduction of quantum gravimeters can not only help improve the data that scientists receive from such sensors but can also change where these sensors are able to be used as well.
As Holynski and colleagues demonstrate in their work, these sensors can be used beyond the lab in outdoor settings which will make the technology much more applicable to fields such as archaeology and civil engineering, which could help save costs as well as potential damage to hidden underground artifacts or utilities.
What they did – To test the sea-legs of their sensor, the team wheeled it roughly 55 yards (50 meters) across the street from the university’s mechanical workshop to a quiet Birmingham road with buildings on either side and occasional traffic rumbling by. Three feet beneath this ordinary road sat a roughly 6.5 x 6.5-foot cross-section of a utility tunnel.
With 10 minutes of data collected from this real-world environment, the team was able to construct a gravity map that pinpointed the underground tunnel using data from the sensor alone. While this measurement doesn’t quite match the sensitivity of measurements made in laboratory conditions, it is nonetheless an accomplishment in the portability and practical use of such a sensor.
What’s next – This work is just the beginning of what this sensor has to offer, Holynski says. In the future, the team has plans to further refine this technology, including making it portable enough to be carried by a single person and testing it on moving platforms.
Holyskin says the team is also interested in how the techniques developed for this sensor can be used to peer into space as well, such as investigating dark matter and gravitational waves.
Abstract — The sensing of gravity has emerged as a tool in geophysics applications such as engineering and climate research, including the monitoring of temporal variations in aquifers and geodesy. However, it is impractical to use gravity cartography to resolve metre-scale underground features because of the long measurement times needed for the removal of vibrational noise. Here we overcome this limitation by realizing a practical quantum gravity gradient sensor. Our design suppresses the effects of micro-seismic and laser noise, thermal and magnetic field variations, and instrument tilt. The instrument achieves a statistical uncertainty of 20 E (1 E = 10−9 s−2) and is used to perform a 0.5-metre-spatial-resolution survey across an 8.5-metre-long line, detecting a 2-metre tunnel with a signal-to-noise ratio of 8. Using a Bayesian inference method, we determine the centre to ±0.19 metres horizontally and the centre depth as (1.89 −0.59/+2.3) metres. The removal of vibrational noise enables improvements in instrument performance to directly translate into reduced measurement time in mapping. The sensor parameters are compatible with applications in mapping aquifers and evaluating impacts on the water table, archaeology, determination of soil properties and water content, and reducing the risk of unforeseen ground conditions in the construction of critical energy, transport and utilities infrastructure, providing a new window into the underground.
Update 02/24 12 p.m. Eastern time: An earlier version of this story stated that this is the first atomic gravimeter capable of taking measurements outside of a mechanics lab. It has since been corrected.
This article was originally published on