Protecting your data in the future will be down to this mind-boggling tech
New research has uncovered ways to bring quantum states to everyday electronics.
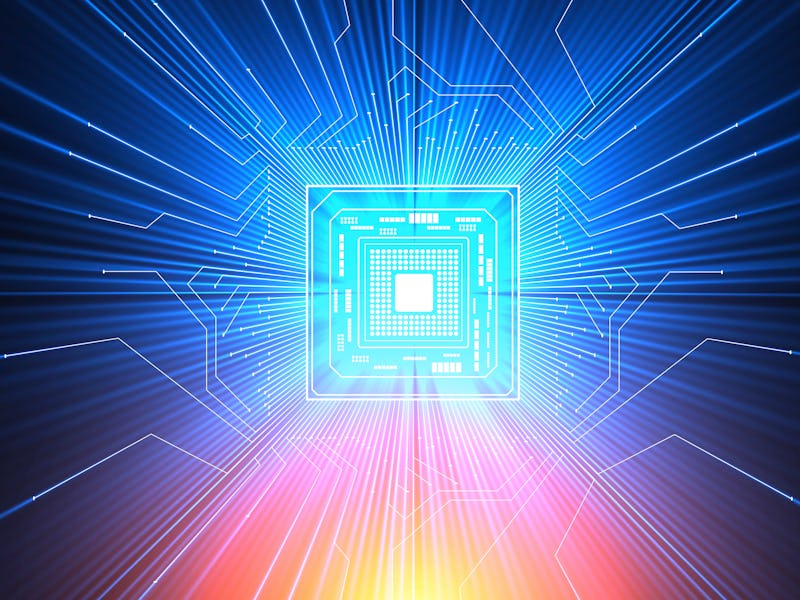
It seems that quantum mechanics is all the scientific rage these days, and consumer electronics could finally be joining it. As electronics approach their miniaturization limits, innovating into the quantum realm, which deals with physics on the atomic level, will be essential for continued growth.
Harnessing quantum mechanics for the next general of technology is a complex and at times elusive goal. But, if done right, it can offer computational power far beyond anything a classical computer can do. This technology has previously been sequestered to the world of supercomputers, but new research has uncovered a way to bring this approach to our everyday electronics as well.
The research, which was recently published as two papers in the journals Science and Science Advances investigated the properties of a wafer-thin material called silicon carbide and how it could be used to control capricious quantum states. From the two studies, the University of Chicago team determined that could not only tune the silicon carbide’s quantum states using run-of-the-mill electric fields but that the quantum states of silicon carbide uniquely emitted photons conducive for communications.
David Awschalom, the team’s lead researcher and Liew Family Professor in Molecular Engineering at UChicago, said in a statement that this exciting quantum property of silicon carbide could pave the way for future quantum communication channels.
“This makes them well suited to long-distance transmission through the same fiber-optic network that already transports 90 percent of all international data worldwide,” said Awschalom.
(From left) graduate students Kevin Miao, Chris Anderson, and Alexandre Bourassa conduct quantum experiments on their silicon carbide.
Controlling the quantum states of materials itself is not a new concept, but such experiments have previously focused narrowly on extremely pure materials like doped diamonds or superconducting metal. This was done primarily to mitigate impurities which could potentially disrupt the materials’ quantum states. A benefit of this study, its first author, Kevin Miao, said in a statement, is that it demonstrated how such control could be achieved with a much more accessible material.
“All the theory suggests that in order to achieve good quantum control in a material, it should be pure and free of fluctuating fields,” said Miao, “Our results suggest that with proper design, a device can not only mitigate those impurities, but also create additional forms of control that previously were not possible.”
While these results are still very far from commercializing such tech, the authors write in their Science paper that they hope their discoveries will play a part in enabling the next generation of quantum technology. Specifically, Awschalom said in the statement that these discoveries could play a role in advancing and securing the world’s communication networks.
“This work brings us one step closer to the realization of systems capable of storing and distributing quantum information across the world’s fiber-optic networks,” Awschalom said. “Such quantum networks would bring about a novel class of technologies allowing for the creation of unhackable communication channels, the teleportation of single electron states and the realization of a quantum internet.”
As data — especially communications data — become increasingly important parts of our geopolitical landscape, continuing to innovate the way we transport and protect such data will be crucial to protecting the privacy and rights of millions of people worldwide.
Abstracts:
Science:
Spin defects in silicon carbide have the advantage of exceptional electron spin coherence combined with a near-infrared spin-photon interface, all in a material amenable to modern semiconductor fabrication. Leveraging these advantages, we integrated highly coherent single neutral divacancy spins in commercially available p-i-n structures and fabricated diodes to modulate the local electrical environment of the defects. These devices enable deterministic charge-state control and broad Stark-shift tuning exceeding 850 gigahertz. We show that charge depletion results in a narrowing of the optical linewidths by more than 50-fold, approaching the lifetime limit. These results demonstrate a method for mitigating the ubiquitous problem of spectral diffusion in solid-state emitters by engineering the electrical environment while using classical semiconductor devices to control scalable, spin-based quantum systems.
Science Advances:
Interfacing solid-state defect electron spins to other quantum systems is an ongoing challenge. The ground-state spin’s weak coupling to its environment not only bestows excellent coherence properties but also limits desired drive fields. The excited-state orbitals of these electrons, however, can exhibit stronger coupling to phononic and electric fields. Here, we demonstrate electrically driven coherent quantum interference in the optical transition of single, basally oriented divacancies in commercially available 4H silicon carbide. By applying microwave frequency electric fields, we coherently drive the divacancy’s excited-state orbitals and induce Landau-Zener-Stückelberg interference fringes in the resonant optical absorption spectrum. In addition, we find remarkably coherent optical and spin subsystems enabled by the basal divacancy’s symmetry. These properties establish divacancies as strong candidates for quantum communication and hybrid system applications, where simultaneous control over optical and spin degrees of freedom is paramount.