Microbes help solve a central mystery of ancient ocean temperatures
This will help researchers better study how ocean temperatures have changed over time.
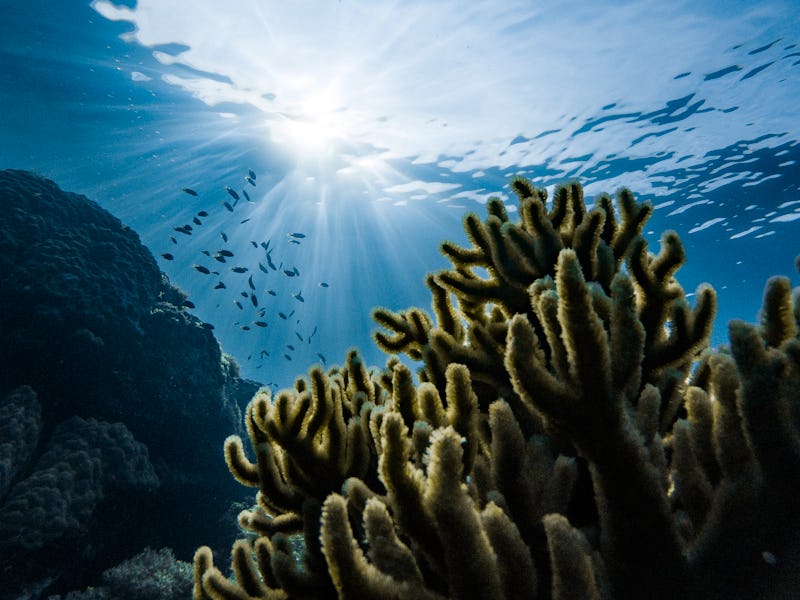
Neither bacteria nor animal, archaea are single-celled organisms that scientists turn to when looking for clues about ocean temperatures deep in the Earth’s past. These little organisms make pretty reliable proxies for changing ocean conditions, allowing scientists to search ancient sediments for clues to changing ocean conditions over time. Now, deeper research into these organisms has given scientists even more confidence in this approach.
The study, published Monday in the journal Proceedings of the National Academy of Sciences, narrowed in on exactly what proteins were at play that caused these organisms to seize up when experiencing changing ocean temperatures and whether or not any other environmental factors may be affecting them as well.
Learning more about the inner workings of these little organisms will help reconstruct “ancient global temperatures and impact our understanding of past temperature variability and Earth system dynamics,” write the study authors, led by Paula Welander, Ph.D., associate professor of Earth system science at Stanford University.
Archaea in sediments have been used to study Rio Tinto, a naturally acidic river.
In this case, the mechanisms in question are related to the archaea’s unique fatty membrane that transforms from a loose, double-layered membrane to a tight, single-layered one under stress, including environmental stressors. The lipids in these membranes are sometimes tightened even more by the addition of hydrocarbon rings along the body. These differences in archaea membranes are important for climatologists because they can be preserved marine sediment and help researchers reconstruct what kind of environment may have triggered the reaction in the archaea.
Researchers previously believed that one particular type of archaea, Thaumarchaeota, has a monopoly on these membrane-modifying rings in the deep ocean as a result of temperature change, but other research had identified archaea in different environments that had similar reactions under stressors like changing salinity and acidity as well. To settle once and for all — at least when it comes to the biome of the Northern Pacific that they tested — the validity of Thaumarchaeota rings as a unique predictor of temperature change, Welander’s team took to the lab.
The team experimented in their lab with an archaea called Sulfolobus acidocaldarius to see what kind of proteins were necessary for it to produce rings. When analyzing and isolating different protein combinations in Sulfolobus acidocaldarius identifyied two unique proteins that appeared to be integral for ring growth, called GrsA and GrsB.
Through comparison with other archaea, the team confirmed that these proteins were only present in deep ocean environments in Thaumarchaeota, meaning the proxy comparison of its rings to oceanic temperature changes was still sound.
Archaea in extreme conditions change their membrane composition, making them show up in unique ways in the fossil record.
“People have been looking for these proteins for 40 years,” Welander said in a related press release. “With that critical information now in hand, we can start to constrain some of the uncertainty about this particular archaea-based paleotemperature proxy.”
Welander says that this discovery will not only give researchers stronger footing when studying these ancient temperature changes but the lessons learned about membrane fusing can also be used in drug discovery and materials science.
“Microbes invent all kinds of weird biochemistry to do all kinds of weird reactions,” Welander said. “Anytime you can expand that chemistry of what is possible, it’s really exciting from just a basic science perspective.”
Abstract:
Archaea synthesize distinctive membrane-spanning lipids (GDGTs) that are readily preserved in ancient sediments and utilized as paleotemperature proxies to reconstruct sea surface temperatures deep in Earth’s past. However, properly interpreting GDGT-based biomarker proxies requires an accurate assessment of the archaea that contribute to GDGT pools in modern environments and of the proteins necessary for synthesizing GDGTs. In this study, we identify 2 radical SAM proteins in Sulfolobus acidocaldarius that are required to produce these molecules. Bioinformatics analyses of these GDGT ring synthesis proteins reveal that Thaumarchaeota are the dominant source of cyclized GDGTs in the open ocean, allowing us to constrain one factor of uncertainty in the application of GDGT-based paleotemperature proxies.