Lab-Grown "Mini-Brains" Emit Brain Waves Similar to Those of Preterm Babies
"We don't know yet how much potential they have."
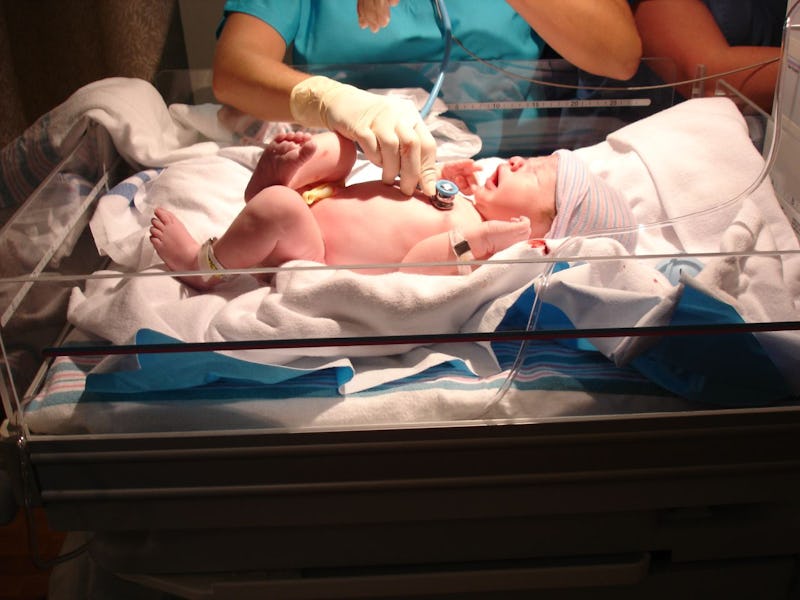
By creating brain organoids or “mini-brains” capable of producing brain waves similar to those of preterm babies, Alysson Muotri, Ph.D., proved himself wrong.
If you had asked the UC San Diego professor five years ago whether a mini-brain could create brain waves similar to those of a full-sized human brain, he would have said no. Now, he tells Inverse, “I think it is inevitable.” After all, his lab-grown mini-brains are already showing a level of neural activity that’s unprecedented in vitro.
Yet Muotri explains that “at the beginning, most people, even myself, were very skeptical about this data.”
For now, his skepticism has been put aside. On Thursday Muotri and his team published their results in the journal Cell Stem Cell, a follow up to a pre-print paper released in 2018. The pea-sized brains, a million times smaller than a typical human brain, that grew in a lab at UC San Diego proved capable of creating bursts of brain waves after existing for about two months. At 10 months, the once-sparse signals appeared more regularly, and at different frequencies.
While these mini-brains grew cellular structures similar to those found in human brains and are the first brain organoid models to develop human-like functional neural networks, the important distinction is that they are human-like and not exactly human. Although there are brain waves, there is no behavior and they lack the sophistication of a human brain network. In the human brain, brain waves underly functions like information processing, perception, and motor coordination.
“But what is the potential of what we have right now?” Muotri asks. “That is what we don’t know.”
The pea-sized brain organoids at 10 months old.
“I get this question a lot: What are they thinking? Are they self-aware? And that is very unlikely,” he continues. “However, the truth is, we don’t have evidence either way. We don’t know yet how much potential they have.”
That’s because, at the moment, scientists don’t have any way to prove whether or not a mini-brain is conscious or not. They are, however, actively attempting to develop some sort of test that could establish consciousness. To get there, Muotri is holding a meeting in October to “discuss the issue of consciousness and brain organoids” with biologists, philosophers, and ethicists.
"I think it is premature to say that they do have something like consciousness — but it might also be premature that they don’t."
For now, it’s intuition that tells Muotri and his colleagues that the mini-brains aren’t capable of consciousness. They are just cells in a dish that are not attached to a body and don’t receive sensory input. But in the end, they still don’t definitively know.
“I think it is premature to say that they do have something like consciousness — but it might also be premature that they don’t,” Muotri allows.
The mini-brains are made from stem cells, and they grow and mature in the same way as the fetal brain once they are placed in a culture that mimics the environment of brain development. In the process, the stem cells differentiate into different types of brain cells, self-organize, and end up as a 3D structure. Over the course of this 10-month study, the team monitored the mini-brains’ neural activities with multi-electrode arrays, which is how they discovered the brain waves.
At two months there were sparse brain waves.
By six months the waves were firing at a higher rate than other brain organoids created before.
And between nine and ten months they had reached a neuroactivity plateau. Despite this plateau, they can actually be alive for several years now — Muotri is currently working on a way to keep the cells inside the organoids from dying, which likely keeps nutrients from reaching the entity’s center.
A cross-section of a brain organoid, showing the initial formation of a cortical plate. Each color is a different brain cell.
In an effort to understand these brain waves better, the team also trained a machine-learning algorithm to record the brain waves of 39 premature babies, all of whom were between 6 and 9-and-a-half months old. That process allowed the algorithm to subsequently predict how old the mini-brains were based on their waves, indicated that the organoids and the fetal human brain grow in a similar matter. They determined that the rhythms of the mini-brains were most similar to those of infants born at 25 to 39 weeks post-conception.
Mini-brains have the potential to revolutionize medical research because they can be used to inform disease modeling and drug screening. Researchers could, for example, create brain organoids that model autism or epilepsy, then use those organoids to examine new treatment options — all without having to test on human subjects.
“As our models improve, we can move toward studying diseases that are complex and perhaps require connections between two brain regions,” Muotri explains. “The potential to do something good for human health is huge.”
And in turn, growing and studying mini-brains teaches scientists about brain evolution. The human cortex is dramatically different from that of the chimpanzee — one of our closest relatives, along with bonobos. What scientists are observing now in petri dishes could reflect what happened at the very early stages of human brain development.
Timing, Muotri says, has proven to be a crucial factor. To learn more, scientists must have the patience to wait, give the cells the right environment, and see how they mature.
Now, they have to figure out what exactly they’ve matured into.
Abstract:
Structural and transcriptional changes during early brain maturation follow fixed developmental programs defined by genetics. However, whether this is true for functional network activity remains unknown, primarily due to experimental inaccessibility of the initial stages of the living human brain. Here, we developed human cortical organoids that dynamically change cellular populations during maturation and exhibited consistent increases in electrical activity over the span of several months. The spontaneous network formation displayed periodic and regular oscillatory events that were dependent on glutamatergic and GABAergic signaling. The oscillatory activity transitioned to more spatiotemporally irregular patterns, and synchronous network events resembled features similar to those observed in preterm human electroencephalography. These results show that the development of structured network activity in a human neocortex model may follow stable genetic programming. Our approach provides opportunities for investigating and manipulating the role of network activity in the developing human cortex.