Latest Gravitational Wave Finding Goes Beyond Einstein
Some of the faintest signals in the universe could unravel its biggest mysteries.
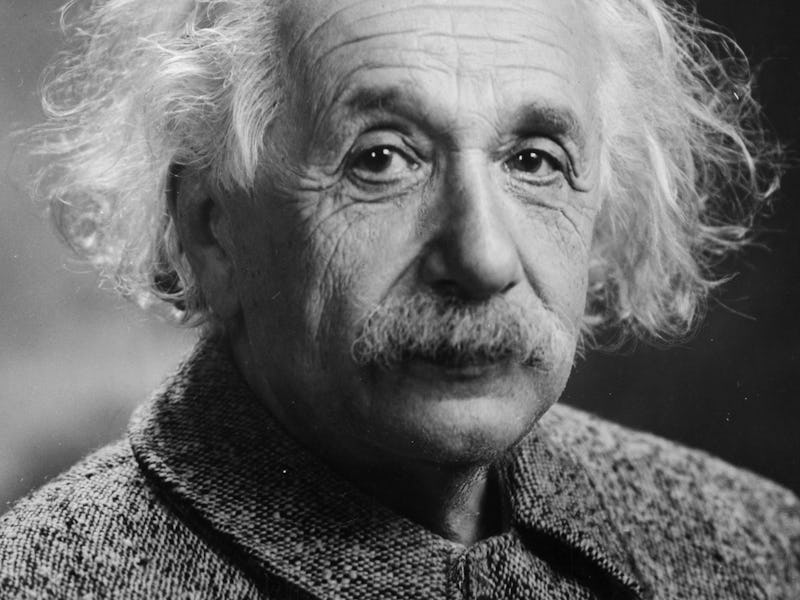
The biggest takeaway from Thursday’s news that scientists have detected gravitational waves for a third time over is three-fold: we’ve discovered a new class of extremely large black holes, we’ve established the efficacy of the Laser Interferometer Gravitational-wave Observatory’s (LIGO) search for these elusive ripples in space time, and we’re beginning to learn much more about the nature and evolution of black holes in ways we never imagined.
And that’s just the tip of the iceberg.
When LIGO has been augmented to a higher state of sensitivity and scientists have a better understanding of how to best pick up gravitational waves, “we should expect to see one binary black hole merger event per day,” said physicist and LIGO researcher Bangalore Sathyaprakash of Penn State and Cardiff University, during a media briefing on Wednesday. Those black hole collisions have produced each of the three major wave events announced in the last 16 months. Those discoveries were groundbreaking their own right: they provide Albert Einstein and his hypotheses that were the backbone of the theory of general relativity correct.
But there is a lot more reason for why we need to continue looking for gravitational waves.
LIGO's third detection of gravitational waves.
“Before our discoveries, we didn’t even know for sure these black holes existed,” said physicist and LIGO scientist Laura Cadonati of Georgia Tech. “What we do know now: they do exist, and they may have played an important role in the early universe. And we’re starting to get a glimpse into how they behave [and] how they possibly could have formed.”
But black holes are just one phenomena that produce gravitational waves powerful enough for our interferometers to detect. The other big tamale physicists want to study are gravitational waves produced by neutron star mergers.
A neutron star is basically the aftermath of a collapse core of a larger star. They are the smallest and densest stars which exist in the known universe — coming out to about 10 to 29 times the mass of the sun (by comparison, the newest black hole merger was about 49 times the sun’s mass). Like black holes, they possess a very unique brand of physical properties which scientists would love to learn more about, and gravitational waves may be key to unlocking those mysteries.
Mike Landry, the head of LIGO’s Hanford, Washington facility, thinks that gravitational waves emanating from the collision of two neutron stars could “tell us about the extreme state of nuclear matter.”
It’s just a matter of actually observing the signals produced by such an event. “We just don’t know how often it will happen” he says. “They are theoretical predictions of that rate, but they are many orders of magnitude uncertain. So we want to measure it with our detectors.”
It’s going to take a lot of luck, but what LIGO’s scientists can do to aid the search is basically work to increase the sensitivity of the interferometers being used to observe such signals. Increasing the sensitivity creates an exponential increase in the accuracy and volume of the area of space the interferometers can search. For instance, increasing the sensitive twice over will increase the volume of the search by two. Increasing sensitivity by a factor of four results in an eight-fold increase in volume.
“That ultimately may be the thing we have to do: just increase the sensitivity of the detector,” said Landry. “Increase the sensitivity, increase the volume of the search, and have a better chance of seeing those first binary neutron star mergers.”
Gravitational waves are just one part of the process, however. Landry explains that observing gamma rays from the same neutron star crashes brings in another sort of data worth analyzing. Combining the insights gained from both sources of data is an example of multi-messenger astronomy — an emerging field where scientists can use multiple assets of measurements to understand the universe more comprehensively.
Landry is hopeful that studying binary neutron star mergers, under a multi-messenger lens — propelled by gravitational waves — could lead to a better understanding of the ‘equation of state of nuclear matter,’ which essentially refers to a succinct way to explain nuclear physics. “It’s the holy grail of physics,” he says.
Cadonati’s own “dream signal,” on the other hand, are waves produced by galactic supernovae. She believes that combined with an analysis of the neutrinos emitted by supernovae and a deeper look at the electromagnetic signals created by such structures, scientists might finally have a better understanding of the physics in a supernova event.
Meanwhile, Sathyaprakash wants to see three big questions answered through gravitational waves: where is gravity from, what does matter with extremely high densities (like from neutron stars) look like, and what is the history of the universe? “These are the deep questions of the origin of the universe the we will be able to answer with gravitational waves,” he said.
For David Shoemaker, the scientific lead for the current LIGO test run, an ideal scenario would mean that the instruments pick up a signal “that no one has predicted and for which no one has an explanation. I want to really surprise the world with a discovery that requires theoretical astrophysicists to go back and start from scratch and think of some new idea for what generated the signal.”
To solve those questions, LIGO is going to need more help. Apart from additional renovations which will increase the sensitivity of the two U.S.-based instruments, we need more interferometers. And lo — there are some bulls here to join the parade.
First up is Virgo, a European interferometer being built near Pisa, Italy. Jo van den Brand of VU University Amsterdam explained on Wednesday that “the most obvious contribution” behind making Virgo operational “is we gain between one and two orders of magnitude in accuracy” when it comes t looking for gravitational waves.
The other big advantage, however, is that “if you were to look at the two LIGO detectors, you realize that they are oriented in the same manner,” he said. “Virgo has a different orientation,” which might allow it to understand other characteristics about the waves it observes.
Another interferometer is being built in India with direct help from LIGO. According to Shoemaker, the design of the two LIGO interferometers is “destined to go into an infrastructure which India is building. The timing for that is determined by the work that’s required to actually establish a new observatory in India.” LIGO is working with Indian engineers in the building of the observatories. The current plan is for that interferometer to go online in 2024. “When it comes online it should be as sensitive as the two LIGO instruments and the Virgo instrument.”
There’s also another instrument coming online in Japan, underneath a mountain. “That one will come in a gradual sort of way,” said Shoemaker. “I think we can say sometime in the mid-2020s, we should have quite a network of detectors.”
After one more confirmed detections of a gravitational wave signal LIGO will start to share its data more publicly with the rest of the world’s scientists. The idea “is to move towards ‘open data,’” said Shoemaker. It will take years before LIGO will be able to expediently upload data collected by its instruments the way an institution like NASA does with its telescopes. “Processing our data is always going to take months.”
Overall, there are few areas of physics more exciting right now than gravitational wave research. “This is informing our astrophysics understand of the universe. It’s really opened a new window of the universe,” said Cadonati. “All the pieces could really start coming together.”