The NuSTAR Telescope Is Rapidly Changing What We Know About the Universe
Lead scientist Daniel Stern talks through the latest breakthroughs.
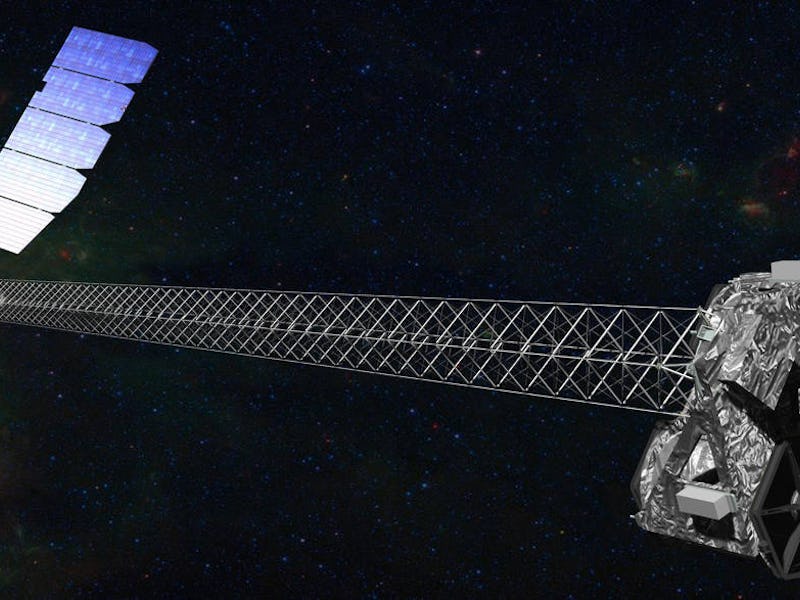
NASA launched the Nuclear Spectroscopic Telescopic Array (NuSTAR) with a Pegasus XL rocket in 2012. Since then, it’s been a force to be reckoned with, shedding light on astrophysics debates that have been simmering for years. This telescopic array, which operates in the X-ray spectrum but with much higher resolution and sensitivity than older orbiting telescopes that work at comparable energies like Swift and INTEGRAL, offers scientists the opportunity to glimpse black holes and other astrophysical phenomena that emit electromagnetic radiation in the X-ray regime (wavelength between 0.01 and 10 nanometers) with unprecedented clarity.
NASA JPL’s Daniel Stern has been the project scientist for NuSTAR since before the array ever launched. He says that NuSTAR picks up where other orbiting X-ray telescopes left off and takes their efforts a few steps — or leaps — further.
“We’re the first mission to focus those X-rays with real imaging optics, whereas previous missions used something called a coded-mask aperture,” Stern tells Inverse. This older technology weighs much more than NuSTAR’s focal equipment — heavier equipment means it costs more to launch — and yields images that aren’t as sharp. “So NuSTAR had this fundamental change in the technology which bought us about a factor of 200 improvement in sensitivity and a factor of more than 10 improvement in sharpness of the images — and actually at a cheaper price tag on top of that. A factor of 200 gain in sensitivity is immense. It just opened up this window on all sorts of things.”
This window has opened on some surprising and impressive discoveries in the five years since NuSTAR’s launch, upending ideas and settling debates. We asked Stern about the most surprising and important.
NuSTAR helped scientists understand what happens inside a star right before it explodes.
NuSTAR Settled How Supernovae Happen
Stars explode all the time. The universe basically started as hydrogen and helium, which condensed into stars, which then fused these elements into heavier metals. When these stars exploded, they formed even heavier metals, like titanium; these materials eventually formed planets.
“Everything in us — from planets to people, from the metal in your jewelry to the silicon in your computer — all was forged within stars, and then spread out into the universe in stellar explosions,” says Stern, echoing Carl Sagan’s famous maxim. Here’s the thing, though: Before NuSTAR, scientists didn’t know what happened inside a supernova in the moments right before it exploded.
It’s been long established that a star’s fusion reaction, which pushes outward, in combination with its gravity, which pulls its material inward, create a dynamic balance. When a star is out of material to burn, it collapses on itself then bounces out, which we know as a supernova. But Stern says that theoretical models for supernovae simply couldn’t account for the strength of the explosion. Computer models would stall shortly after the bounceback.
Two competing theories come to play here: Either an extra source of energy was breaking the stall or the star’s spin created a jet that would break the stall. Stern explains that one of the fundamental designs of NuSTAR was that it would be able to study titanium — which, as we know, gets formed during the supernova explosion — and the energetics and the position of the titanium would be the way to test between these two models. So in 2014, the scientists studied Cassiopeia A, a well-observed supernova in the Milky Way that blew up about 500 years ago, to find out who was right.
“We thought it was gonna be one or the other, that we would either see a ring of titanium if the whole explosion had extra energy and went out, or maybe we’d see a line of titanium from a jet along the spin axis of the supernova,” says Stern. But they didn’t see either. Instead they saw blobs of titanium. From this evidence, along with new theoretical models developed by other science teams, NuSTAR scientists were able to conclude that the material inside of a star “sloshes” around inside, creating enough energy to break the stall and explode.
“It was this exciting hand-in-hand with the theorists and the observers,” recalls Stern. “We came to the same answer around the same time. So it knocked off this more than 20-year hole in our understanding of the universe: how do stars explode?”
Other X-ray telescopes had approached this same question, but not until NuSTAR’s high-precision imaging came along were scientists able to get a clear picture. “They were all trying to do fudgy fingerprints, and we were able to do DNA testing,” says Stern.
NuSTAR helped scientists rule out the "obscuring cloud" model of iron line distortion(bottom) in favor of the "rotation" model (top), proving that we can indeed measure black hole spin.
NuSTAR Can Measure How Fast Black Holes Spin
For the 15 years leading up to NuSTAR’s deployment, X-ray satellite telescopes had provided ambiguous measurements of black holes. It wasn’t clear whether the material accreting around black holes was obscuring the X-ray emissions coming out from the objects. This uncertainty allowed scientists to use the evidence provided by X-ray emissions from iron in a black hole’s accretion disk to draw very different conclusions. And while the two main models of black hole spin matched the observations made by low-energy X-ray telescopes, they had very different predictions of what would occur at higher energy levels.
Then NuSTAR came along and started detecting high energy X-ray radiation. Lo and behold, it cleared up the mystery, settling the debate: The black hole at the center of NGC 1365 spins just under the maximum speed that Einstein predicted. And more importantly, this helped scientists understand that X-ray emissions from black holes were not being obscured by the clouds of material around the black holes but by the immense gravitational distortion caused by the black holes.
“Most people now agree that we’re measuring how fast black holes are spinning,” says Stern, “and we’re often finding them spinning close to the maximum speed that they’re allowed to spin.”
NuSTAR data collected near a supernova in the M82 "Cigar Galaxy" helped scientists identify the first known ultra-luminous pulsar.
NuSTAR Discovered the First Ultra-Luminous Pulsar
In 2014, some college students at University College London were having a star party on the roof.
“They looked up at M82, the Cigar Galaxy, and there was a new star in it,” says Stern. “The students realized it wasn’t in their textbook. This was the nearest type Ia supernova to go off in 150 years.”
According to Stern, nearly every terrestrial and orbiting observatory started collecting data on this unexpected supernova, named 2014J. Since NuSTAR’s preliminary mission was to study supernovae, and since it was still a pretty small mission that could afford to devote much more time to a single observation than other large observatories could, the NuSTAR team trained the telescope on the supernova for about a month. What they saw, besides the supernova, was a brightly emitting X-ray source in M82’s galactic disk.
NuSTAR scientists used high-energy X-rays coming from the pulsar (pink) to identify it.
It had long been a mystery what this and other similar bodies on the edges of galaxies, so-called ultra-luminous X-ray sources, were. Some scientists suspected that they were intermediate-mass black holes, weighing around a thousand times as much as the sun. These small black holes would be accreting matter at typical rates, right below what’s known as the Eddington Limit. Below the Eddington Limit, luminosity and gravity are in harmony, and the black hole only throws off large amounts of material when accretion jumps above the limit. Others thought these ultra-luminous X-ray sources could be small black holes, like the kind formed in a supernova, which were accreting material well above the Eddington Limit.
But when timing analysis expert Matteo Bachetti looked at the data collected by NuSTAR, he found that the object was pulsating like a heartbeat. This ruled out a black hole, since pulsation requires a strong magnetic field. For Stern and his colleagues, this confirmed that the object was a neutron star.
“And then given how luminous it was, it had to be accreting at hundreds of times the Eddington Limit,” he explains. Thus the NuSTAR team helped find and identify the very first ultra-luminous pulsar. And while this doesn’t prove that every ultra-luminous X-ray source is a pulsar, it does prove that this one is, as are the two that have been discovered since then. Theorists are still a bit lukewarm on the discovery, but Stern says these objects are providing valuable data on what super-Eddington accretion looks like.
“The physics gets very weird when you’re above that speed limit, and it’s something that we think is important for black hole growth early in the universe,” he says. “It’s really hard to study 10 billion light-years away what super-Eddington accretion onto a super massive black hole is like, but from these nearby systems we can get really great data and try to understand the physics.”
This is one depiction of what a black hole's corona might look like. NuSTAR might help confirm this in coming years.
What Will NuSTAR Discover Next?
Stern hopes that the next thing NuSTAR will help clear up is the shape of a black hole’s corona. The corona is a formation of high energy plasma that surrounds a black hole. Stern says that as a black hole’s accretion disk throws off optical and ultraviolet light, the corona augments their energy to create X-ray radiation.
“We’re pretty confident that most of a black hole’s X-ray emission starts off when this plasma, which we call the corona, upscatters the thermal photons from the accretion disk.”
In what should now come as no surprise, there are three competing theories for what a corona looks like. One is that it’s a ball that completely surrounds the black hole, one predicts that it looks like an atmosphere that sandwiches the accretion disk like an Oreo, and one is that the corona looks like a jet above and below the black hole, along its spin axis.
“Right now there are various observations that point to different ones of these models being correct, but there’s no consensus yet,” admits Stern. He hopes that if the first five years of the NuSTAR mission are any indication of what’s to come, in the next few years, we should at least know what a black hole’s corona looks like. After all, says Stern, we’re making startling astrophysical discoveries all the time.
“I think we’re in a golden age right now for sure.”