These Super-Dense Stars Have Something Strange Lurking In Their Cores
The heaviest neutron stars probably have cores made of quarks.
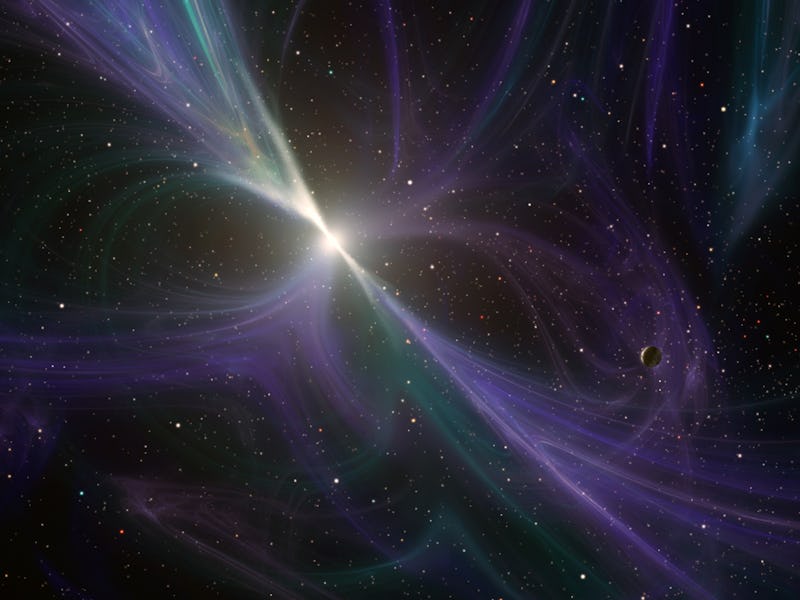
Some neutron stars probably have something strange lurking in their cores, according to a recent study.
Neutron stars are already some of the most peculiar objects in the universe. They contain enough mass to make up two or three of our Sun, except it’s all crammed into a space just a few miles wide. All that matter is packed in so tightly that its atoms have been crushed and then squashed into neutrons (subatomic particles with no electric charge, one of the building blocks of atoms).
Under that tremendous pressure, physics can get pretty weird. Some physicists have theorized that the cores of some neutron stars could contain an extremely bizarre type of matter, one made of tiny subatomic particles called quarks (which are the building blocks of more familiar particles like neutrons and protons).
A recent study published in the journal Nature Communications, concluded there’s about an 80 to 90 percent chance that some neutron stars really are dense enough to squash neutrons into quarks at their cores.
Some neutron stars, called pulsars, emit beams of radiation from their magnetic poles as they spin.
Physics Gets a Little Quarky
University of Helsinki physicist Eemeli Annala and his colleagues calculated the probability that the physical processes deep inside a neutron star could lead to a ball of quarks. To come to this conclusion, they input everything astronomers and astrophysicists currently know about neutron stars, supernovae, and quarks into a supercomputer. Then, they asked the supercomputer to calculate the probability of different outcomes, given all of that information.
According to the results, the likelihood of neutron stars with quarky cores is around 80 to 90 percent.
Normally, quarks don’t just exist on their own. You can’t go fill up a bucket of quarks, for example. Quarks tend to form bonds with other subatomic particles called gluons, and together, they combine to form protons and neutrons (which, along with electrons, form the atoms that make up everything except neutron stars, black holes, and some other cosmic weirdness, that is.) But under the extreme pressure present at the center of a massive neutron star, there’s not enough room for neutrons to keep being neutrons. They get crushed into their constituent bits, meaning quarks and gluons.
The result is an exotic type of matter that may not exist anywhere else in the universe: cold quark matter (not to be confused with cold dark matter, which is different and even weirder). It’s called cold quark matter because there is, in theory, another way to make quark matter: extreme heat, which can basically melt neutrons and protons down into free-floating quarks.
Likely, But Not Definite — Yet
For the most massive neutron stars — ones with two to three times the mass of our Sun — the less likely alternative to a core of quark matter is a black hole. According to Annala and his colleagues’ calculations, there’s about a 10 to 20 percent chance that, instead of squashing neutrons into quarks at the center of the neutron star, that process might just destabilize the whole thing. That could trigger a final collapse into a black hole.
The deciding factor seems to be exactly how neutrons under pressure turn into quarks. Specifically, how much energy is involved and how smooth the transition is. Annala and his colleagues say gravitational waves may hold the answer. Sooner or later, gravitational wave observatories like LIGO should get a chance to measure the ripples in space-time caused when two neutron stars merge, and those waves should carry more information about what’s going on inside the two dense stellar corpses.
Understanding more about whether — and how — neutron stars crush neutrons into quarks at their centers could shed light on what happens deep inside the cooling corpses of stars whose mass hovers at the boundary between forming a neutron star or a black hole. It could also shed light on how the universe itself works, from the enormous scale of massive stars exploding to the tiniest scale of quarks and gluons.