NASA finally knows what is beneath the surface of Mars
“The fact that we recorded it confirmed that the core is liquid.”
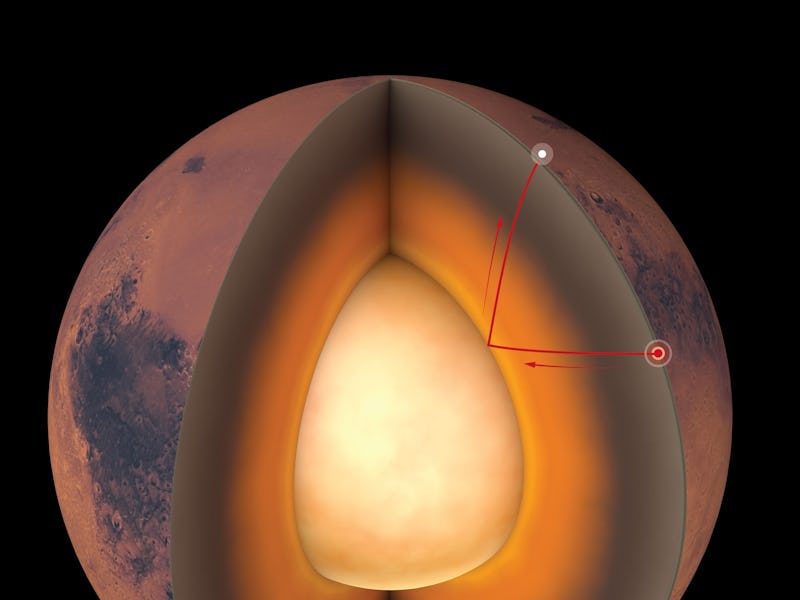
If you’ve ever taken a planetary science course, or maybe just cracked open a book on the Solar System, you may have seen diagrams of the internal structure of the planets, showing their crusts, mantles, and cores.
What you may not realize, NASA Jet Propulsion Laboratory geophysicist Mark Panning tells Inverse, is that those diagrams “are cartoons and guesses,” based on gravitational measurements. The only planet whose structure scientists actually understand in detail is Earth.
We know the innards of Earth through seismology measurements — something that hasn’t been available for other planets. Until recently, this was true: According to a trio of papers published Thursday in Science, researchers can finally confirm Mars has a large liquid metal core.
Panning is the JPL project scientist for the NASA InSight mission, which landed a seismometer on the Red Planet in 2018 and has been recording Marsquakes ever since. NASA's InSight (Interior Exploration using Seismic Investigations, Geodesy and Heat Transport) lander was designed to reveal the inner structure of Mars through seismological and thermal readings taken from a landing site on Elysium Planitia, a plain along the Martian equator.
Panning and colleagues used InSight seismometer data to establish the thickness and possible structure of the Martian crust, the temperature of the planet’s mantle and make the first direct seismological confirmation of Mars’ core.
“This goes from cartoons to our actually knowing what the inside looks like,” he says.
What’s new — In the study of Martian crust, researchers used readings of two kinds of seismic waves — P-waves and S-waves — to determine the potential structure and thickness of the crust.
P-waves are faster than S-waves, Panning says, and measuring the difference in their arrival times at the InSight seismometer allows researchers to locate a Marsquake.
Importantly, when a P-wave crosses a physical transition, such as from the mantel to the crust, “most of the energy continues on as a P-wave, but a little bit gets turned into an S-wave,” Panning says. Measuring the difference in timing of those two waves tells researchers when the waves have crossed a layer.
This region of Mars is called Cerberus Fossae. This is where the two largest quakes detected by InSight likely originated.
The researchers saw three “bumps” in the resulting data that can be explained equally well by two models.
In one model, the crust beneath InSight has two layers and is about 20 kilometers thick. In the other, the crust has three layers and is about 39 kilometers thick.
Extrapolated across the planet, this suggests the Martian crust averages between 24 and 72 kilometers thick, and “both of those are actually on the thin end of our pre-mission expectations,” Panning says. Some models had previously put the crust at 100 kilometers thick.
Meanwhile, the study examining Mars’s mantle found the structure looked at seismic waves bouncing off the surface multiple times to arrive later than P- and S-waves, Panning says, and measured their velocity to understand the consistency and temperature of the mantle.
“In order to match the velocities we observed, you have to keep the mantle relatively cool compared to the range of models we considered before we had data,” he said.
The findings mesh with the results of the crustal study since a hotter mantle under a thinner crust would result in more volcanism than is seen on Mars today, Panning added. The cooler mantle observations also drove the researchers’ estimate that the Martian crust is richer in heat-producing radioactive elements — by a factor of 13 to 20 — than the mantle.
Illustration of Mars’ internal structure.
The Martian core study used ScS waves — S-waves bouncing off the core — to define the core’s radius of around 1,800 kilometers while also confirming gravity measurements that suggested the core was liquid.
“A solid core would not have produced a strong enough reflection for us to have seen it,” Panning says. “The fact that we recorded it confirmed that the core is liquid.”
How they did it — The core measurements were the most difficult to obtain, Panning says, and scientists needed multiple measurements to confirm the tiny ScS-waves were real.
“None of these events are big. The biggest event we’re looking at is maybe a magnitude 3.8,” he says. “That’s a size that in California where I am, there’s a really good chance you wouldn’t even feel it, depending on how close you are.”
The measurements were made possible first by the extreme sensitivity of the seismometer, which Panning says can measure vibrations on the scale of a hydrogen atom, but also due to the measurements being done on a dry planet like Mars.
“On Earth, there’s always seismic noise that a seismometer records the oceans drive that,” he says. “Our seismometer on Mars is probably two orders of magnitude quieter than the best station on earth.”
Why it matters — Earth is really why research on the deep interior of Mars matters.
Earth is a rocky planet, as are Mercury, Venus, and Mars, and understanding the formation and evolution of other rocky planets is key to understanding the one we call home.
“If you want to be a doctor and you only study one patient, you’re not going to be a very good doctor,” Panning says.
What’s next — The next steps for Panning and collegues will be to dig deeper into the data they have now, but with InSight scheduled to keep operating into 2022, they are also continuing to record Marsquakes.
If they get lucky enough to measure some quakes at different ranges than their current measurements, it might even allow them to resolve whether the two or three-layer model of the Martian is correct.
“The story is not over,” he says. “We have to wait and see.”
Martian Crust Study abstract: A planet’s crust bears witness to the history of planetary formation and evolution, but for Mars, no absolute measurement of crustal thickness has been available. Here, we determine the structure of the crust beneath the InSight landing site on Mars using both marsquake recordings and the ambient wave field. By analyzing seismic phases that are reflected and converted at subsurface interfaces, we find that the observations are consistent with models with at least two and possibly three interfaces. If the second interface is the boundary of the crust, the thickness is 20 ± 5 kilometers, whereas if the third interface is the boundary, the thickness is 39 ± 8 kilometers. Global maps of gravity and topography allow extrapolation of this point measurement to the whole planet, showing that the average thickness of the martian crust lies between 24 and72 kilometers. Independent bulk composition and geodynamic constraints show that the thicker model is consistent with the abundances of crustal heat-producing elements observed for the shallow surface, whereas the thinner model requires greater concentration at depth.
Martian Mantle Study abstract: For 2 years, the InSight lander has been recording seismic data on Mars that are vital to constrain the structure and thermochemical state of the planet. We used observations of direct (PandS) and surface-reflected(PP, PPP, SS, andSSS) body-wave phases from eight low-frequency marsquakes to constrain the interior structure to a depth of 800kilometers. We found a structure compatible with a low-velocity zone associated with a thermal lithosphere much thicker than on Earth that is possibly related to a weak S-wave shadow zone at teleseismic distances. By combining the seismic constraints with geodynamic models, we predict that, relative to the primitive mantle, the crust is more enriched in heat-producing elements by a factor of 13 to20. This enrichment is greater than suggested by gamma-ray surface mapping and has a moderate-to-elevated surface heat flow.
Martian Core Study abstract: Clues to a planet’s geologic history are contained in its interior structure, particularly its core. We detected reflections of seismic waves from the core-mantle boundary of Mars using InSight seismic data and inverted these together with geodetic data to constrain the radius of the liquid metal core to 1830 ±40 kilometers. The large core implies a martian mantle mineralogically similar to the terrestrial upper mantle and transition zone but differing from Earth by not having a bridgmanite-dominated lower mantle. We inferred a mean core density of 5.7 to 6.3 grams per cubic centimeter, which requires a substantial complement of light elements dissolved in the iron-nickel core. The seismic core shadow as seen from InSight’s location covers half the surface of Mars, including the majority of potentially active regions—e.g., Tharsis—possibly limiting the number of detectable marsquakes.
This article was originally published on