Scientists detect ancient cosmic 'background noise' hidden in old Arecibo observatory data
These faint ripples in space-time are billions of years old.
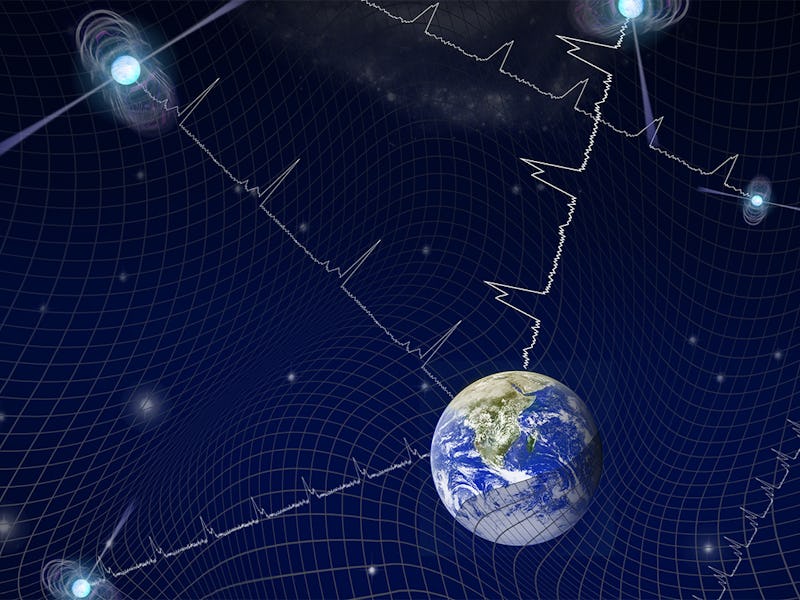
Over the course of 13 years, a consortium of astronomers have been observing the signals from rotating stars in the hopes of catching faint ripples in space-time, known as gravitational waves.
Finally, they may have possibly found hints of the first low-frequency gravitational waves ever detected.
The announcement came from a team of researchers from the North American Nanohertz Observatory for Gravitational Waves (NANOGrav), who say they have discovered a faint signal that may be caused by low-frequency gravitational waves, the first of its kind. Remarkably, these data come from the now defunct Arecibo Observatory.
The new discovery is detailed in a study published in the January issue of The Astrophysical Journal, and has major implications for how we observe — or rather, listen to — the universe.
Here's the background — On September 15, 2015, scientists made the first detection of a signal from gravitational waves. The signal was the resulting ripple from a merger between two black holes that had collided 1.3 billion years ago.
The first gravitational waves detected were a result of two massive black holes colliding together, creating a ripple through space-time.
Gravitational waves are caused by the accelerated masses of cosmic beings, which send out waves at the speed of light. If electromagnetic radiation, or light, is a way for astronomers to see the universe, gravitational waves are a way to listen in to the universe.
Using the Laser Interferometer Gravitational-Wave Observatory (LIGO) detectors and the Virgo detector, scientists are able to detect the signals created by these waves.
By the time they reach Earth, the gravitational waves are relatively weak and last for mere seconds. Scientists analyze the signals and trace the origin of the gravitational waves, listening in to the events of the early universe.
The majority of the these events captured by the detectors are caused by the collision of two black holes.
Low-frequency gravitational waves are different. They are created over billions of years by large numbers of black holes circling one another, emanating signals with much longer wavelengths.
Compared to their high-frequency counterparts, low-frequency gravitational waves are more persistent background noise.
As a result, they require years of data to be detected.
How they did it — NANOGrav is made up of more than 100 astronomers from across the United States and Canada united by the single quest to detect low-frequency gravitational waves.
The astronomers used a type of star known as a pulsar to make their discovery. Pulsars are compact stars with a very strong magnetic field, and they rotate while emitting a beam of electromagnetic radiation. Due to their unique qualities, scientists often use pulsars as 'cosmic lighthouses.'
Pulsar stars are known as the universe's time keepers, emitting light at a regular timing. Therefore, any irregularities in that timing may have possibly been caused by gravitational waves stretching and shrinking space-time, according to the study.
The ripples caused by gravitational waves cause slight deviations in the time expected for the pulsar signal to arrive on Earth, indicating that the position of Earth has shifted.
The researchers observed a group of pulsars using the Arecibo Observatory in Puerto Rico, which collapsed in December 2020, ending its reign as one of the largest radio telescopes in the world.
They measured the timing of the signals being emitted by the pulsars scattered across the sky at the same time, a technique known as a “pulsar timing array,” and were able to detect minute changes in the Earth’s position from the stars.
What they found — This is the strongest signal yet of a low-frequency gravitational wave. The scientists cannot yet confirm that the minute changes are the result of a low-frequency gravitational wave signal. But they did rule out other possibilities for these changes, such as interference from other matter in the Solar System.
“It is incredibly exciting to see such a strong signal emerge from the data,” Joseph Simon, a member of NANOGrav and lead researcher of the study, said in a statement.
“However, because the gravitational-wave signal we are searching for spans the entire duration of our observations, we need to carefully understand our noise," he adds.
"We can strongly rule out some known noise sources, but we cannot yet say whether the signal is indeed from gravitational waves. For that, we will need more data," he says.
What's next — This detection took years in the making, but it may take the scientists at NANOGrav a few more years to confirm it was indeed low-frequency gravitational waves that caused the deviation in Earth's position from the pulsar's signal.
To do that, the researchers will have to expand the dataset, adding more pulsar stars and observing them for even longer periods of time.
At the same time, they also need to rule out other possible causes for the space-time ripple. The team at NANOGrav are producing computer simulations to test whether the detected noise was caused by something other than gravitational waves.
"Trying to detect gravitational waves with a pulsar timing array requires patience," Scott Ransom, a researcher at the National Radio Astronomy Observatory and the current chairperson of NANOGrav, said in a statement.
"We're currently analyzing over a dozen years of data, but a definitive detection will likely take a couple more. It's great that these new results are exactly what we would expect to see as we creep closer to a detection."
Whether or not this discovery is confirmed as the first low-frequency gravitational waves, scientists are definitely keeping a closer ear to the cosmos.
Abstract: We search for an isotropic stochastic gravitational-wave background (GWB) in the 12.5 yr pulsar-timing data set collected by the North American Nanohertz Observatory for Gravitational Waves. Our analysis finds strong evidence of a stochastic process, modeled as a power law, with common amplitude and spectral slope across pulsars. Under our fiducial model, the Bayesian posterior of the amplitude for an f−2/3 power-law spectrum, expressed as the characteristic GW strain, has median 1.92 × 10−15 and 5%–95% quantiles of 1.37–2.67 × 10−15 at a reference frequency of the Bayes factor in favor of the common-spectrum process versus independent red-noise processes in each pulsar exceeds 10,000. However, we find no statistically significant evidence that this process has quadrupolar spatial correlations, which we would consider necessary to claim a GWB detection consistent with general relativity. We find that the process has neither monopolar nor dipolar correlations, which may arise from, for example, reference clock or solar system ephemeris systematics, respectively. The amplitude posterior has significant support above previously reported upper limits; we explain this in terms of the Bayesian priors assumed for intrinsic pulsar red noise. We examine potential implications for the supermassive black hole binary population under the hypothesis that the signal is indeed astrophysical in nature.