Scientists weigh one of the most mysterious particles in the universe
By getting a neutrino mass, we can fine tune our understanding of the universe around us — and maybe find hints to dark matter.
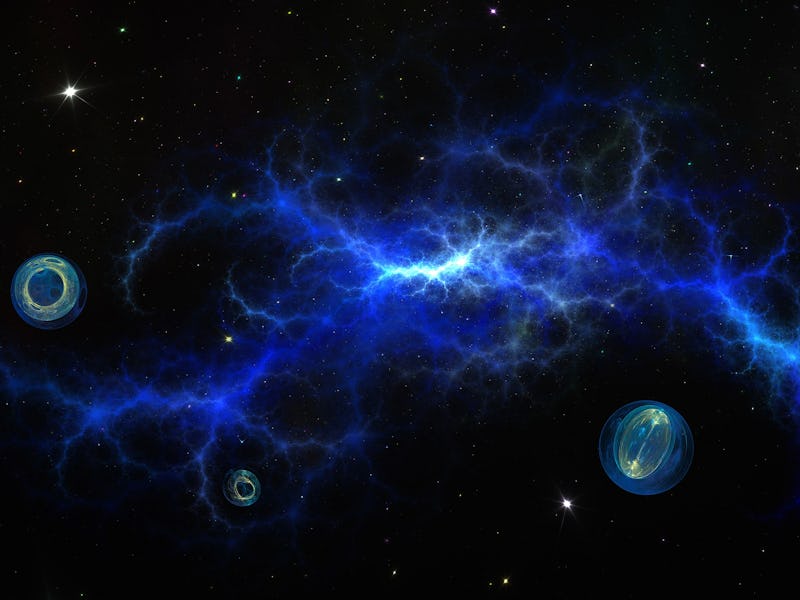
Neutrinos may be the most mysterious particles in the universe. These ghostly entities zip around at nearly the speed of light and can fly through matter easily — a light-year's worth of lead would only stop about half of the neutrinos flying through it. They are so light that no experiment to date has successfully weighed them.
Now scientists have come the closest yet to pinpointing the mass of neutrinos — they have discovered the electron antineutrino is less than one-billionth of a proton's weight. The scientists detailed their findings online Monday in the journal Nature Physics.
WHAT ARE NEUTRINOS? — Of all the known particles that have mass, neutrinos and their antimatter counterparts are the lightest and the most numerous. Both come in three known "flavors" — electron, muon, and tau.
Neutrinos and antineutrinos are generated by nuclear reactions and the radioactive decay of unstable atoms. They are electrically neutral and only rarely interact with other particles — roughly a thousand trillion neutrinos pass harmlessly through your body every second.
For decades, the Standard Model — currently the best explanation for how all the known elementary particles behave — assumed that neutrinos had no mass. Unexpectedly, around the turn of the millennium, researchers discovered neutrinos don't just pick one flavor and stick to it, but can switch from one identity to another, a change that could only happen if neutrinos had mass. The discovery won the Nobel Prize in Physics in 2015.
WHY IS FINDING THE NEUTRINO'S MASS IMPORTANT? — The fact that neutrinos have mass revealed the Standard Model cannot be the complete theory of the fundamental particles of the universe, hinting at enticing discoveries beyond the Standard Model.
Although neutrinos are light, their combined weight is estimated to be roughly equal to the weight of all visible stars in the universe taken together. This suggests their gravitational influence should have played a key yet still-uncertain role in the evolution and structure of the universe.
Many other puzzles exist concerning neutrinos. For example, it remains unknown if neutrinos and antineutrinos are distinct particles, or whether neutrinos may be their own antiparticles. It is also uncertain whether more flavors of neutrino may exist, ones that could explain the dark matter that researchers think may make up roughly five-sixths of all matter in the universe. It's not even known which of the three flavors neutrinos is the lightest, or if neutrinos get their mass the same way as other particles with mass.
A key step in solving all these mysteries is pinpointing features such as the neutrino's mass. But knowing the neutrino has mass is not the same as actually measuring it.
The KATRIN main mass spectrometer.
WHAT DID SCIENTISTS FIND? — Researchers have now discovered the mass of the electron antineutrino is less than 1.6 * 10^-33 grams or 0.8 electronvolts. Einstein's famous equation E=mc^2 revealed that mass is equivalent to energy — 0.8 electronvolts is 80 percent of the energy a single electron gains when accelerated by one volt in a vacuum.
This is the first time that a neutrino weighing experiment has confirmed that neutrinos are of sub-electronvolt mass, as long suspected. "Of course, it would have been more surprising and more exciting if we actually found the neutrino mass, but what we found was an upper limit, which is probably what everyone expected," study co-author Magnus Schlösser, an astroparticle physicist at the Karlsruhe Institute of Technology in Germany, tells Inverse.
WHAT DID THEY DO? — The researchers experimented with a radioactive form of hydrogen known as tritium. Whereas normal hydrogen atoms lack any neutrons in their nuclei, tritium atoms each have two neutrons.
Tritium's unstable nature often leads one of its neutrons to decay into a proton, emitting an electron and an electron antineutrino. Since this electron and electron antineutrino share the 18,600 electronvolts of energy released from the decay, measuring the energy of the electron can in turn reveal the electron antineutrino's mass.
The KATRIN experiment at the Karlsruhe Institute of Technology in Germany houses the world's most intense source of tritium, generating 95 billion tritium decays per second. Superconducting magnets steer electrons from tritium's decay through a giant 75-foot-long, 200-ton spectrometer to measure their energy with unprecedented resolution, making KATRIN the world's most sensitive scale for neutrinos to date.
"The size and complexity of the instrument are impressive," experimental particle physicist Angelo Nucciotti at the University of Milano-Bicocca in Italy, who did not take part in this research, tells Inverse. "When I think of the complexity of KATRIN and how they succeeded in getting everything under control, I am amazed."
KATRIN also had to account for as many sources of uncertainty as possible that might undermine the accuracy of the results, Schlösser says, such as the interactions of the electrons with the tritium. Previous experiments that failed to do so led to nonsensical results, such as neutrinos with imaginary mass traveling faster than the speed of light.
"The success of this measurement relied on the very precise understanding of every component," Nucciotti says. "Every component had to be improved with respect to the state of the art, and its behavior had to be understood with unprecedented precision. They had to develop special instruments to characterize all the components of the experiment. Really a huge effort, which has been perfectly and successfully orchestrated."
WHAT'S NEXT? — These initial findings resulted from roughly two months of measurements. When KATRIN finishes its planned 1,000 days of measurements, scientists hope to pinpoint the electron antineutrino's mass to as low as 0.2 electronvolts. "Its full potential has not yet been exploited," Nucciotti says.
Abstract — Since the discovery of neutrino oscillations, we know that neutrinos have non-zero mass. However, the absolute neutrino-mass scale remains unknown. Here we report the upper limits on effective electron anti-neutrino mass, mν, from the second physics run of the Karlsruhe Tritium Neutrino experiment. In this experiment, mν is probed via a high-precision measurement of the tritium β-decay spectrum close to its endpoint. This method is independent of any cosmological model and does not rely on assumptions whether the neutrino is a Dirac or Majorana particle. By increasing the source activity and reducing the background with respect to the first physics campaign, we reached a sensitivity on mν of 0.7 eV c–2 at a 90% confidence level (CL). The best fit to the spectral data yields m2 ν = (0.26 ± 0.34) eV2 c–4, resulting in an upper limit of mν< 0.9 eV c–2 at 90% CL. By combining this result with the first neutrino-mass campaign, we find an upper limit of mν< 0.8 eV c–2 at 90% CL.