Self-healing “Xenobots” mean a future with living machines
“Biological machines” redefine what scientists consider life.
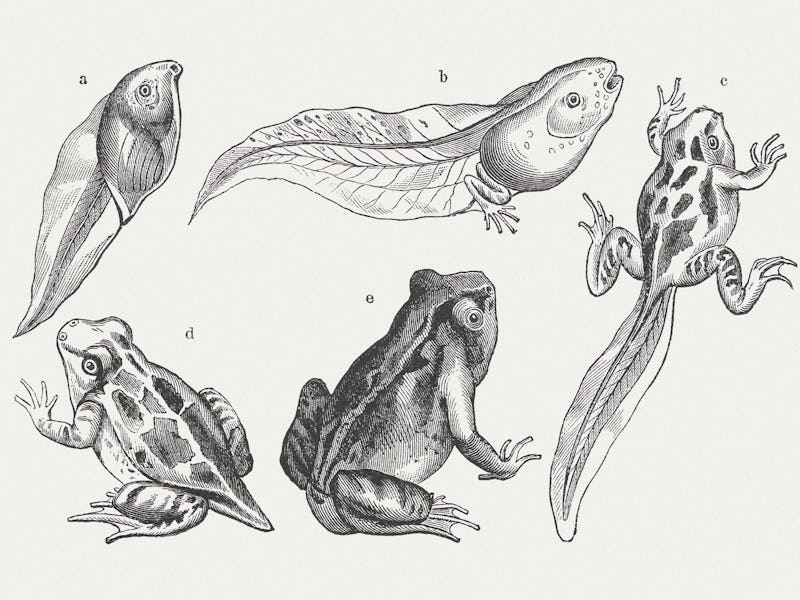
One hundred years ago, it was easy to tell when something was a machine. Machines were “hard and clanky, metallic, and pretty heavy,” as developmental biologist Michael Levin tells Inverse.
But lately, it’s become clear to Levin and his collaborator Joshua Bongard, a professor of computer science at the University of Vermont, that our definitions of “machine” and “living organism” are about to get really, really murky.
In January of 2020, the two first made headlines after announcing their team had successfully created “completely biological machines.” Now, Levin and Bongard have taken their biological machines, or “Xenobots,” to the next level — using frog cells to create life forms capable of motion, memory, and manipulation of the world around them.
These incredible results were published Wednesday in the journal Science Robotics.
The background — The 2020 biological machines were designed in computer simulations, then built in Petri dishes using micro-scalpels and living biological tissues — skin and muscle cells harvested from embryos of the African clawed frog, Xenopus laevis. In other words, they’re designed like one would design a robot, but the raw materials are 100 percent living cells.
The Xenobots were designed to exhibit “swarm” activity.
Levin and Bongard landed on a design reminiscent of the dog-turned-ottoman from Beauty and the Beast that, once carved into shape, would actually contract its muscle cells and walk across the dish. They called their living creations Xenobots (pronounced zenno-bot), after the frog that donated its cells.
“Already in that first paper we showed some creatures whose evolutionary history took place in a computer,” Levin, a professor at Tufts University, says.
“They had an evolutionary history, it just wasn’t on Earth. It was in this completely virtual world that Josh Bongard coded up.”
A few weeks ago, Bongard published his new theory on whether it’s helpful to still use the metaphor that living things are like machines (spoiler: It’s not). In the team’s latest bot advance, there’s no sculpting needed. The new Xenobots aren’t ottomans, they’re spheres. And they don’t have muscle cells, they’re all skin. But they still move around just fine.
What’s new — The skin of a Xenopus frog is covered in cilia, tiny hairlike structures, which redistribute mucus across their skin. It’s an adaptation to keep bacteria and fungi at bay. When you put embryonic skin cells together, they conglomerate into little spheres with the cilia on the outside.
The team found that the cilia on this new Xenobot “work in concert so the thing can go forward,” Levin says. “They start to row and the thing moves.”
The Xenobots exhibited what the scientists call cooperative activity.
The researchers also tested to see if they could set the Xenobots up with a form of rudimentary memory. They injected them with RNA that encodes a protein that changes color when it’s exposed to a certain color of light. It worked: The bots made the proteins, and it was clear which bots had “seen” the light when they came back a different color.
“It’s a proof of principle that we can modify the cells to put in other things that give them new functionalities,” says Levin. “Synthetic biology nowadays is all ‘cell soups,’ it’s all cells in culture. Now they can be embodied — we have a body into which you can put all of these kinds of circuits.”
The team was also interested in how the Xenobots would act as a group. Bongard ran more computational simulations to try and predict what would happen when you let the bots into an arena with a bunch of particles. How would the bots’ motion redistribute the particles? Can you get them to make them into piles?
It was an iterative process, measuring properties of the Xenobots, putting the data into the computer simulation to make predictions, then testing those predictions on the bots and measuring again. “It goes simulation, experiment, simulation, experiment, and hopefully each time we’re a bit smarter after each go-round,” Levin says.
Why this matters — Practically speaking, Xenobots could have applications like swimming through the bloodstream and unclogging arteries. But Levin is more excited about the big picture.
“Most of the problems of modern medicine would go away... if we understood how to make cells build whatever we want them to build.”
When Xenobots grow over their short 10-day lifespan, they elongate and become transparent.
“They almost look like ghosts,” Levin says. “And it’s nothing you would have predicted, they don’t look like frog embryos, they don’t look like tadpoles. They have their own brand-new developmental sequence.”
It’s a whole new type of model organism, Levin explains, that will help us understand how cells build structures, and how we can “motivate them” to make different things besides their genetic default — no genetic engineering required.
This video breaks down the steps it takes to create a Xenobot.
“Most of the problems of modern medicine would go away… if we understood how to make cells build whatever we want them to build,” he says.
When probed about whether it was just an accident that this configuration of ciliated cells can move, Levin pushed back at the question. He says a lot of people don’t take evolution seriously enough.
“Our own complex cognitive properties evolved from humbler, simpler versions of those exact same properties in other [earlier] organisms,” he says.
“[Are the Xenobots] using physics to repurpose their mechanisms in a way that’s explainable by chemistry and physics? Of course they are. There’s no magic. But it’s the same for us. When we walk around, we’re using the electricity of electrical networks in our brains to activate our muscles. That doesn’t mean it’s any less wondrous.”
“People are very binary in things. They say is it a robot, or is it an organism? Yes and yes,” says Levin. “These binary classifications are just no good anymore.”
What’s next? — Levin and his team are moving forward with their experiments, including some using cells from sources beyond frogs. He’s driven by his curiosity about how groups of cells work together to form a sort of collective intelligence — just like our human brains.
“That’s a problem that’s kept me awake since I was a kid,” he says. “How does the structure of the body relate to the mind that lives in there somehow?”
As these living bots get more and more complex, society will have to grapple with what it means to be cognitive. Levin imagines a future straight out of a science fiction movie — one that will challenge how we view cognition, and what we ethically owe our creations.
“In your lifetime, we’re going to be surrounded by just an incredible plethora of new agents that are weird hybrids and cyborgs and robots with living tissue embedded and [vice versa] — every combination under the sun is going to be running around somewhere,” says Levin.
Abstract: Robot swarms have, to date, been constructed from artificial materials. Motile biological constructs have been created from muscle cells grown on precisely shaped scaffolds. However, the exploitation of emergent self-organization and functional plasticity into a self-directed living machine has remained a major challenge. We report here a method for generation of in vitro biological robots from frog (Xenopus laevis) cells. These xenobots exhibit coordinated locomotion via cilia present on their surface. These cilia arise through normal tissue patterning and do not require complicated construction methods or genomic editing, making production amenable to high-throughput projects. The biological robots arise by cellular self-organization and do not require scaffolds or microprinting; the amphibian cells are highly amenable to surgical, genetic, chemical, and optical stimulation during the self-assembly process. We show that the xenobots can navigate aqueous environments in diverse ways, heal after damage, and show emergent group behaviors. We constructed a computational model to predict useful collective behaviors that can be elicited from a xenobot swarm. In addition, we provide proof of principle for a writable molecular memory using a photoconvertible protein that can record exposure to a specific wavelength of light. Together, these results introduce a platform that can be used to study many aspects of self-assembly, swarm behavior, and synthetic bioengineering, as well as provide versatile, soft-body living machines for numerous practical applications in biomedicine and the environment.
This article was originally published on