New atomic clocks could herald in a "second quantum revolution"
The next-generation atomic clocks could bring breakthroughs from fundamental physics to navigation.
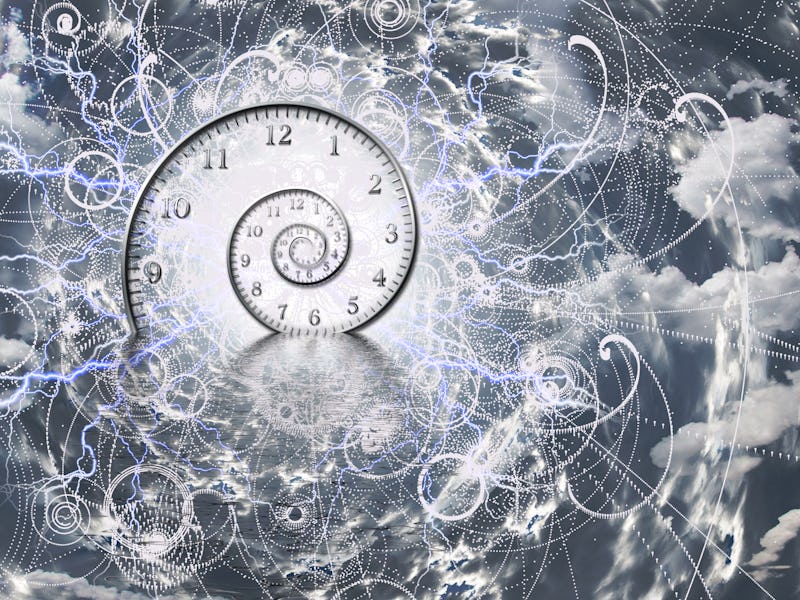
Next-generation atomic timekeeping techniques could push new frontiers in quantum physics, according to a new study.
Atomic clocks are essential elements in GPS and other navigation systems — and a group of scientists laid out a new blueprint for an atomic clock that’s so precise it could spur a second revolution in quantum science. Smarter than the typical atomic clock, the idea is the first to use an algorithm paired with a quantum computer to stamp out noise in fine measurements.
This study is “just the first step,” though a significant one, towards the goal of a next-generation level atomic clock, Peter Zoller, theoretical physicist at the University of Innsbruck in Austria, tells Inverse. Zoller led the theory behind the study, published in Nature today.
What’s new — What the collaboration has envisioned and built is a quantum sensor that is essentially a piece of an atomic clock — though it will be another challenge to build it into a functioning clock. The team used what’s called a trapped ion quantum computer to make an ultraprecise sensor that shows how much better atomic clocks could be. Zoller likens this to building a nice new engine for a car — exciting, but to really see what it can do, you have to install it first. “The second step has not happened yet,” he says, but he hopes the idea will catch on and have an impact among professional atomic clock researchers.
“I think it’s very exciting work,” Jun Ye, a physicist at JILA joint institute between the National Institute of Standards and Technology and the University of Colorado who wasn’t involved with the study, tells Inverse.
The algorithms Ye chooses to pursue going forward may not be precisely the same, but he’s excited to explore the underlying idea behind Zoller’s paper. He also believes this kind of “quantum optimization” will drive the new frontier of quantum science.
Here’s the background — Atomic clocks are so precise because they are based on the fundamental properties of a certain type of atom contained within, and these properties don’t change. However, they rely on a laser that interacts with those atoms, and the laser needs to be kept at a very precise frequency, fixed by the atoms. As tiny imperfections in the clock cause the laser to drift from the ideal frequency, a device called an interferometer tracks this deviation so that the clock can get back on track.
The quantum sensor Zoller and his colleagues built is a type of interferometer made up of 26 ions. What’s special about it is the fact that it’s programmable — following an algorithm designed to make it as sensitive as possible.
If you want to make more accurate measurements, you need more atoms in your interferometer— though there’s a limit to how many you can manage, Zoller says. Typically, these atoms act independently of one-another, but “quantum mechanics allows correlation between atoms” — called entanglement, which is the source of quantum computing’s power. Einstein famously called this “spooky action at a distance.”
This strange quantum property can be harnessed as a kind of hack to make quantum computers much more powerful than regular ones. It also makes a huge difference for quantum sensors too.
The difference in sensitivity between typical interferometers and entangled ones is about the difference between the square root of the number of atoms in the system and the number of atoms, Zoller says. For example, that means with 10,000 atoms the new method beats the old with a sensitivity 100 times greater.
Currently, no operating atomic clocks rely on this principle, Zoller says, but the time has come “to add entanglement to the story.” The team harnessed entanglement, though not for quantum computing. They used it instead, “in order to make better measurements” in atomic clocks, Zoller says.
Specifically, they did this by using the quantum computer to run an algorithm to make their quantum sensor better. “We would like to suppress the noise in the interferometer as far as we can,” Zoller says.
Why it matters — The reason they need a fancy, quantum machine to run this algorithm is so that it can tweak the knobs controlling the experiment to cut through the noise and make the best sensor possible.
It’s possible to choose both what type of entanglements to create between the particles, and choose what properties to study. The algorithm “plays around with the entanglement, plays around with the measurement,” Zoller says, then finds the combination that will result in the most sensitive measurement and uses those parameters.
Before this algorithm, experimentalists didn’t have much they could do. “You could not optimize,” Zoller says. You just took a certain number of atoms, and you dealt with the noise that you got from them.
The computer optimizes both what goes into the system and what gets measured coming out of it. The algorithm asks “what is the best measurement that I can do?” Zoller says, and this process allows you to approach the fundamental physical limits of how good a quantum sensor can be.
More than a fashion statement — Atomic clocks are “already so accurate” that one might ask what there is to gain with more sensitive instruments. They will help make GPS and space navigation more precise, but there’s more to atomic clocks than wayfinding.
“Today, we can build atomic clocks that are so accurate, that if I raised the atomic clock by one millimeter,” you could measure how the change in its surroundings affected the passage of time it experienced due to Einstein’s theory of general relativity, Zoller says.
What’s next — Using this level of precision, the possibilities are endless. For example, an aircraft equipped with an atomic clock might be able to detect the subtle gravitational changes that give away a hiding submarine beneath the waves, or natural resources under the Earth, Zoller says.
This precision will also let scientists ask some very fundamental questions about science. Zoller is most excited about using these ridiculously precise tools to test out gravity itself, specifically in trying to solve one of the biggest challenges in physics — reconciling the seemingly incompatible fields of quantum mechanics and gravity.
Algorithms like Zoller’s might make atomic clocks precise enough to measure the effects of gravity on the micron scale, Ye says, small enough to probe the fundamental properties of gravity.
Scientists are just finding the entrance to a world of new physics in building “a new generation of quantum sensors,” Zoller says.