This beautiful math equation could herald a quantum computing breakthrough
The Fibonacci sequence may unleash the potential of nascent quantum computers.
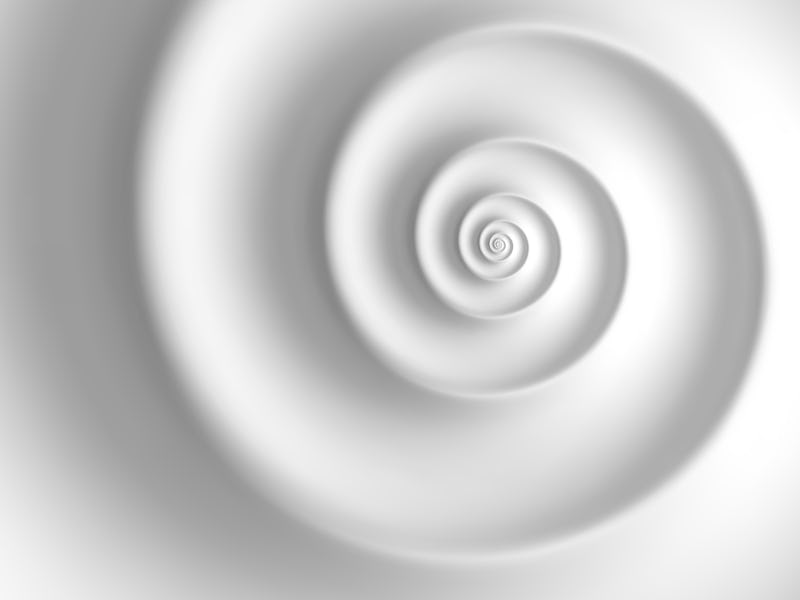
At first glance, the Fibonacci sequence might seem like no more than a bit of mathematical trickery. But look around, and it pops up again and again and again, in computer science and in nature. It’s elegant logic: Add the two previous elements to get the next one.
Now, physicists have applied that logic to yet another setting: the inside of a quantum computer. With the help of a laser, they’ve created a new phase of matter that switches states to a Fibonacci-like rhythm in time. The results, published in Nature on June 20, mark the first time a phase quite like this has been created in the real world.
What’s new — This new phase consisted of ten ions of ytterbium, a rare earth element that’s in quite common use in quantum computers, caged in an electric field.
The ions are only one part of the story. The other is a laser that the scientists fired at the ions to manipulate them. The researchers could flip them, to a regular beat, between one of two states. Call them A and B.
What made the phase unique was the pattern it followed. Instead of a straight periodic pattern, the researchers added a second layer in the style of the Fibonacci sequence. So, instead of an A-B-A-B-A-B pattern, they put the ions through something like A-AB-ABA-ABAAB-ABAABABA, each segment the sum of the two before.
The Fibonacci sequence, sometimes called the golden ratio, appears over and over in nature. (This illustration does not depict nature.)
Over the past several years, the theoretical physicists behind the work had ironed out the theory of how this phase worked. They had modelled and simulated it on their own (classical) computers. But there was no substitute for the real thing.
“The real [quantum] computers are very complicated devices that have many sources of errors that you don’t necessarily anticipate,” Philipp Dumitrescu, a theoretical physicist formerly at the Flatiron Institute in New York City and the paper’s lead author, tells Inverse.
So, they turned to a quantum computer operated by Quantinuum (a spinoff of engineering conglomerate Honeywell), situated in the Denver suburb of Broomfield, Colorado. Inside that quantum computer’s cold heart, they got the phase to work as they expected.
Here’s the background — The qubit is the building block of a quantum computer. A qubit is analogous to the bits that lie at the heart of normal, or classical, computers; they can be a “zero” or a “one.” But because of the vagaries of quantum mechanics, a qubit can be a whole rainbow of combinations of those two states: what physicists call quantum superposition.
Quantum computers probably won’t replace the world’s work machines and gaming rigs anytime soon. But some scientists think they could be valuable for cryptography and pharmaceutical discovery. Physicists have a much more personal use: By using qubits to simulate other computers, they can perform quantum experiments that aren’t really possible in another setting.
While nearly every classical computer in existence uses bits of silicon, quantum computers today use all sorts of different things as their qubits. Some use photons. Some use electrons. Some use atomic nuclei. Some use little contraptions made from superconductors. Some, like the one in this experiment, use ytterbium ions. As long as something has two states to stand in as a “zero” and a “one” respectively, and as long as that thing could be in a superposition of those two states, it could theoretically work as a qubit.
Quantum computers, like those at the Leibniz Computing Center (pictured), require their qubits to exist in a state of “superposition” — both 1 and 0 rather than one or the other.
Why it matters — One of quantum computing’s major challenges is that qubits are delicate. They sit at a precarious precipice, liable to losing their information thanks to interference for all sorts of seemingly minute outside noise: interactions with other particles, even vibrations from heat (it’s why most quantum computers need to be chilled at temperatures barely above absolute zero.)
So physicists are working out how to make qubits more resilient in the face of randomness. One way they can do that is to place the qubits in a certain phase. “There are special types of phases of quantum matter which have ‘protected’ quantum information,” says Dumitrescu. “Those phases of matter can cancel out all sorts of errors.”
That’s precisely what this new phase of matter does. Continually manipulating the qubits with a laser can bolster the qubits, making them hardier and longer-lasting. Using not one but two patterns, thanks to the Fibonacci sequence, can give the qubits an extra layer of protection. Until now, Dumitrescu, that hadn’t been tried in a quantum computer.
The researchers’ results reflected that. Under a quasi-periodic laser, the ytterbium ions at the edge of the qubit cluster lasted over three times longer than the same ions in periodic pulses.
What’s next — This experiment brought together theoretical physicists with their experimental quantum-computer-tinkering counterparts. For the former, it was a vindication of their calculations.
But for the quantum computer folks, the work has just begun. If they want to use this phase in their operations, they'll need to create new algorithms and new routines that work with the new phase. And there may be other phases like this one, which demonstrate quasi-periodicity, that they may want to play with.
“There’s still a lot of interesting work and research to be done,” says Dumitrescu.