Inside the scientific quest to future-proof corn, America's favorite side dish
Taking cues from cacti and orchids, scientists are trying to hack corn’s natural abilities.
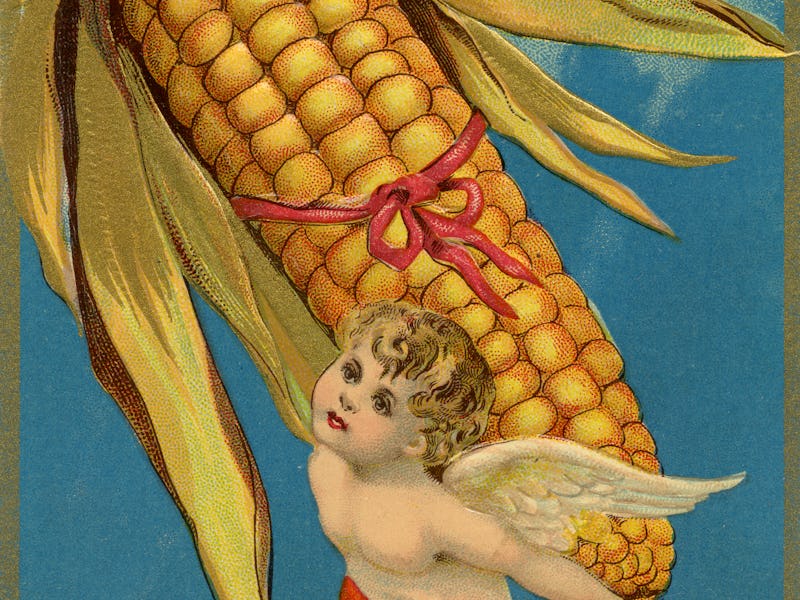
This past summer, a widespread drought across the United States lowered crop yields by as much as one-third as corn, wheat, barley, and other plants suffered from too much heat and too little water. It’s a scenario that will likely become more common as climate change makes much of the world a hotter, drier place.
Scientists are trying to teach old crops some new tricks that will let them flourish in these harsher conditions — turning to secrets that reside in plants like pineapples, orchids, and agaves. These and certain other plants have hacked photosynthesis in ways that allow them to thrive when it’s hot and dry and even to withstand blistering periods of drought.
Many orchids, for example, live in nooks and crannies of trees where their only water comes in sporadic bouts of rain, while others, like agaves, thrive in the rocky soils of desert grasslands. If scientists could engineer crop plants like rice and wheat to be more like these heat-tolerant species, crops could be grown in lands that can’t be farmed right now. Under the right conditions, researchers say, some crop yields could increase by 50 percent or more.
The work is still years from being done, but it could be vital. Climate change is predicted to cause more droughts and make farmland less productive. At the same time, the number of people the world needs to feed will increase to 10 billion from 8 billion by the end of the century.
“It is getting more and more apparent that climate change is going to be a big challenge,” says Xiaohan Yang, a plant molecular biologist at Oak Ridge National Laboratory in Tennessee. “These plants are a natural solution to mitigate climate change.”
The trouble with photosynthesis
Orchid biology could help future-proof crops like corn.
Traditionally, crop improvements have come from targeting traits like the size of the plant, its resistance to pests, or the length of its growing season. But in recent years, scientists have been targeting photosynthesis, the process by which plants grow that ultimately fuels almost all life on Earth.
Photosynthesis uses sunlight, water, and carbon dioxide to make sugars and other molecules plants need. But in dry or hot environments, the dual requirements for water and carbon dioxide present a dilemma: To let carbon dioxide in, plants must keep open small pores on their leaves. But those same pores also let water vapor out. When it’s hot and dry, that can lead to deadly water loss, inefficient photosynthesis, or both.
Photosynthesis happens in two main stages, however, providing an opening for scientists to work with. In the first part of photosynthesis, called the “light reactions,” the plant captures photons from the Sun. The main point of this stage is to create energy-storing molecules that will fuel reactions in the next step. It’s akin to filling up a tank of gasoline so you can be at the ready.
The second stage of the process, the “dark reactions,” doesn’t require light. An enzyme called rubisco grabs carbon dioxide that has entered the leaf and attaches it to a molecule known as RuBP. The sunlight’s energy that was captured and stored earlier is used to fuel reactions that create a simple sugar from the carbon. The plant can use sugars to make more complex molecules.
“within five years, we should have a pretty good idea whether this is going to work or not.”
This version of photosynthesis is how 85 percent of all plants do things, including most trees and most major food crops — rice, wheat, soybeans, and more. Such plants are referred to as C3 plants because they make a three-carbon molecule in one of the first steps of photosynthesis.
Although only the first part of photosynthesis requires light, in most plants, both parts of the process — including grabbing CO2 — happen at the same time while the Sun is shining. If it gets hot, the pores in the leaf either remain open and lose water or close and shut off access to CO2 in the air. If the pores close, the concentration of CO2 inside the leaf drops, so there’s less CO2 for photosynthesis. Worse still, it can really gum up the works — because the enzyme rubisco starts grabbing oxygen instead. This kicks off a wasteful process called photorespiration, during which the plant has to throw out some of the carbon it’s painstakingly collected. Photorespiration can cut the efficiency of fixing carbon by 40 percent, stunting the plants.
Plants have figured out two slightly different ways to get around the problem, and scientists are hoping to exploit both of them. Some plants use a process called Crassulacean acid metabolism, or CAM: They take in CO 2 during the night, while it’s relatively cool, and concentrate and store it until it can be used during the day to make sugars. Other plants — known as C4 plants — concentrate and store carbon dioxide in specialized cells, thus avoiding wasteful photorespiration.
In both cases, these plants have separated the part of photosynthesis that captures carbon dioxide from the air from the part of the process where rubisco grabs the CO2 and begins the process of turning it into sugar. CAM plants separate the processes according to the time of day, and C4 plants separate them physically in different parts of the plant.
The adaptations help plants in two different ways. In the first place, they save water, letting the plant make do with less. Just as important, by limiting the wasteful effects of photorespiration they let the plants grow bigger from the same amount of nutrients.
The CAM strategy
Succulents take in carbon dioxide at night and concentrate and store it until it can be used in the day to make sugars.
CAM got its name from Crassulaceae, the family of succulent plants in which it was first observed. The strategy, with its extra step, evolved starting some 20 million years ago.
CAM plants open the pores in their leaves, called stomata, at night when it’s relatively cool. Then, instead of using rubisco, the CO2-grabbing enzyme that C3 plants rely on, CAM plants use an enzyme called PEP to capture CO2. Unlike rubisco, PEP is very specific to CO2 and won’t take hold of oxygen. The plant then converts the CO2 to a chemical called malate and tucks it away for the night in a cellular closet called a vacuole.
When the Sun comes up, CAM plants can close their stomata to conserve water because they’ve already got carbon stashed away in the vacuole. That carbon can now be converted back to C02 and used by rubisco to build the molecules the plant needs.
Many scientists think CAM is a promising target for engineering. Because CAM evolved independently many times in many different plants, there shouldn’t be a fundamental barrier to inducing the process in non-CAM plants, Katharina Schiller and Andrea Bräutigam write in the 2021 Annual Review of Plant Biology.
In fact, CAM seems to rely on enzymes and other molecular machinery that are already found in C3 plants — they just use them in different ways at different times. That suggests it’s possible to repurpose already existing genes in normal plants to make them CAM plants.
CAM’s complexities
Cacti may hold clues to making corn and other crop plants more hardy.
But that is easier said than done. To make a CAM plant, researchers would have to create biochemical pathways to not only make malate at night but also to transport malate around the cell and then release the CO2 when the time is right.
For now, scientists are still working to understand CAM well enough to control it. That has been painstaking work over many decades, and there are still unanswered questions. Much of today’s knowledge comes from studying the common ice plant (Mesembryanthemum crystallinum), which is able to switch from C3 metabolism to CAM. By studying the differences in the two metabolisms, scientists have been able to figure out many of the processes that have to kick in to make CAM work. And the devil is in the details.
For instance, scientists had identified 13 enzymes and regulatory proteins that seemed to be involved in storing CO2 in the form of malate and then getting it back out again. To better understand the role of each of them, plant molecular biologist John C. Cushman of the University of Nevada, Reno, and his colleagues inserted the genes for each one into a non-CAM plant called mouse-eared cress (Arabidopsis thaliana, the lab rat of plant science). Then they measured how much of a difference each gene made. They also measured where in the cells the regulatory proteins and enzymes were put to work.
Most of the genes involved in malate production would increase malate at least a little bit when turned on one at a time. And most of the ones involved in switching malate back to CO2 would decrease it, the team reported in 2019 in Frontiers in Plant Science.
Cushman and colleagues have also focused on another feature of CAM plants: the thickness of their leaves. Many CAM plants have thick, fleshy leaves, a trait called succulence that helps them retain and store water (think of a cactus stem or the leaves of a jade plant or orchid). The trait appears to be important since succulence seems to make CAM more efficient, helping the leaf retain the stored CO2. Using genes from wine grapes that cause the fruit to become fleshy and ripen, the researchers have increased the succulence of mouse-eared cress, creating leaves that store more water than normal.
With so many complicated mechanisms to be coordinated, there’s still a lot of work to do. Schiller and Bräutigam point out that it’s not enough to know what genes need to be turned on to get production of particular enzymes. The genes also need to be turned on in the right places and at the right times, and produce the right amount of proteins.
“I would say, within five years, we should have a pretty good idea whether this is going to work or not,” Cushman says.
Yang, at Oak Ridge National Laboratory, is optimistic that CAM engineering can work because evolution has come up with the same solution many times independently. Given enough time and effort, he says, synthetic biology and genome editing will be able to replicate the process.
How corn does it
Corn is a staple foodstuff and holiday favorite, but it is not immune to extreme heat.
Another approach to keeping photosynthesis humming along efficiently even when it’s hot and dry is to engineer C4 traits into C3 plants. Many of our cereal crops are already C4 plants, including corn, sugarcane, and sorghum, and evidence suggests that the trait has evolved independently upwards of 60 times. (C4 is named after a characteristic four-carbon molecule produced by the plants during photosynthesis, compared with the three-carbon molecule produced by C3 plants.)
C4 plants also convert CO2 to storage-friendly malate before sending it down the assembly line to make sugars. And C4 plants have evolved a particular leaf anatomy: They pack two types of leaf cells — mesophyll cells and bundle sheath cells — in concentric circles. Carbon dioxide enters the mesophyll cells, as it does with C3 plants. But in C4 plants, the enzyme rubisco is sequestered only in the bundle sheath cells. This arrangement keeps the enzyme surrounded by CO2 and away from oxygen, thus minimizing wasteful photorespiration.
“We feel like we’re sort of on the bleeding edge of the technology development all the time.”
The pores of C4 plants don’t do the open-only-at-night trick, and the plants are generally less water-efficient than CAM plants, although still twice as efficient as C3 plants. Their big advantage is that by sequestering rubisco in the bundle sheath cells, they cut down on photorespiration.
If rice was turned into a C4 plant, “Models predict that yield could increase by 50 percent; water use efficiency would be hugely improved, as would nitrogen use efficiency,” says Jane Langdale, a geneticist at the University of Oxford in the UK who leads the C4 Rice Project, a long-running effort of several research groups funded by the Bill and Melinda Gates Foundation.
Two years ago, researchers with the project introduced five genes from corn into a rice plant. These five, they figured, were the minimum number necessary for the basic reactions: Get the CO2 turned into malate and then back into CO2 again. All of the genes yielded the proteins they were intended to, and the rice plants suffered no harm. What’s more, the altered rice did create malate. But it did not convert the malate back into CO2, and researchers are still trying to figure out why. “That’s the major focus of what’s being looked at, at the moment,” Langdale says.
Still, the work was enough to convince Langdale and her colleagues that they can get portions of C4 metabolism working in rice. At a minimum, they would be happy to have C4 photosynthesis working alongside C3 photosynthesis.
CAM vs. C4 throwdown
Although the C4 and CAM approaches share similarities, they have different strengths and weaknesses. CAM is relatively simpler since you don’t have to arrange the leaf cells in the special C4 way. And because many existing plants have both C3 and CAM characteristics, there’s reason to think that even partial CAM pathways will be a benefit to plants, Cushman says. On top of that, CAM is more water-efficient.
On the other hand, C4 is more likely to create big gains in crop yields while also increasing water use efficiency compared to C3 plants, Langdale says.
“CAM has never evolved to increase yield, or biomass. CAM has evolved as a survival mechanism under severe stress conditions,” she says. “So I don’t think you'd ever want to put CAM in to increase yield. But you may want to engineer CAM to use in marginal lands, for example.”
It’s even possible that you could do both: engineer CAM traits into C4 plants like corn to make them even more efficient at using water, Cushman says.
In both cases, it’s not clear yet whether commercial crop plants are possible. Yang says that it’s clear that CAM can be engineered into C3 plants, but it remains to be seen whether useful crops will result. If they can, he estimates it will be about 10 years before they are available.
“First step is, can we do it? I think yes,” he says. “But then, can we optimize it?... That’s the next question.”
The C4 Rice Project, for its part, gathered momentum in 2006 with the understanding that it would be a long-term effort requiring a lot of basic research. According to the original timeline, it’s not until 2039 that the project expects to hand a working C4 rice plant to commercial breeders.
In the current phase, the researchers are trying to create a prototype of rice that displays basic C4 characteristics, and it’s likely to be four or five years more before they know for sure whether C4 rice will work. They need to figure out how to get the plant to change malate back into CO2, and they would like to be able to increase the size of cells around the veins in the leaf as a step toward specialized bundle sheath cells, among other things.
“It’s really hard to predict. We feel like we’re sort of on the bleeding edge of the technology development all the time,” Langdale says. “And so what that means is, we take two steps forward, one step back, two steps forward, one step back — which is part of the excitement, but also part of the frustration.”
This article originally appeared in Knowable Magazine, an independent journalistic endeavor from Annual Reviews. Sign up for the newsletter.
This article was originally published on