Tiny particle accelerators could help beat back tumors
The " accelerator-on-a-chip," may be small, but it packs a punch.
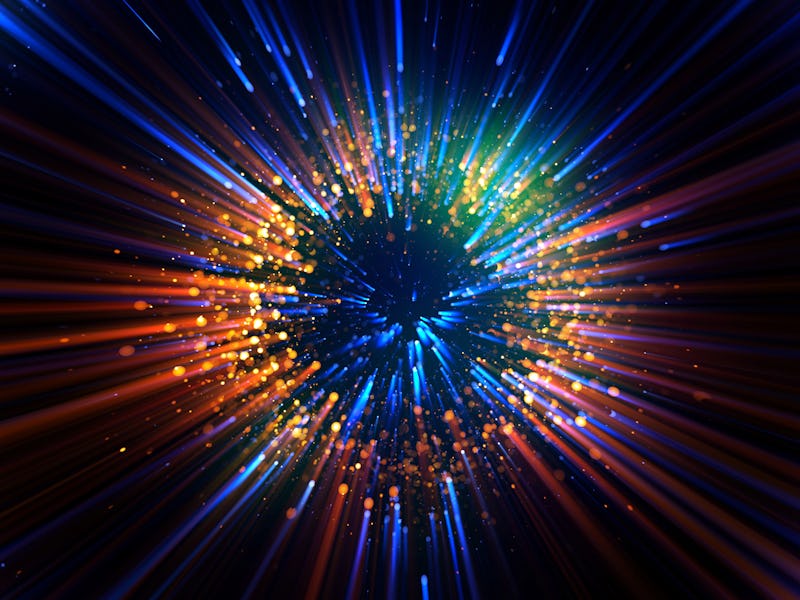
Particle accelerators are typically massive, mile-long electron racetracks, but for the first time scientists have discovered a way to shrink this technology to the width of a human hair to create a particle accelerator on a tiny, silicon chip. While less powerful than their mile-long counterparts, scientists say these miniature accelerators could be used to better target tumor growth in cancer patients.
In many ways, particle accelerators are exactly what they sound like. In traditional, large scale particle accelerators electrons are propelled along long, vacuum-sealed tunnels by microwave radiation to reach speeds very close to the speed of light. This process creates a powerful beam of electrons that researchers can use to examine the atomic and molecular structures of different materials. But, such accelerators are usually tucked away at academic institutions like CERN, Stanford or Fermi Labs and are difficult to scale to human-sized levels.
That’s what the authors of a new study, published Thursday in the journal Science, hope to change. The study describes a proof-of-concept design in which the team etched nanoscale “tunnels” on to a silicon chip and pushed electrons through them using nudges from infrared light.
The study’s first author, Neil Sapra of Stanford, told Inverse that the basic principle behind their miniaturized accelerators and traditional accelerators are largely the same, but that their model’s smaller size also positively affects its cost and scalability by using commercially available lasers.
“The underlying principle is quite similar to traditional accelerators, in that we are using electromagnetic radiation (light) to accelerate particles. In the case of traditional accelerators, they rely on radio-frequency (RF) waves that have a wavelength on the order of centimeters,” Sapra tells Inverse. “In these dielectric laser accelerators, we take advantage of the commercial availability of powerful visible and near-infrared lasers with a wavelength of a micron. In both cases there is the need for ways to guide the radiation, cavities where the acceleration occurs, focusing elements to confine the electron beam, and monitoring elements to determine the state of the electrons. However, with the scale-down of the wavelength we can shrink our accelerator by a similar 10,000 factor!”
This image (magnified 25,000 times) shows a section of a prototype accelerator-on-a-chip that is one-tenth the width of a human. The Rorschach like curves are carefully etched silicon channels designed to guide the infrared beam.
By operating on the nano-scale, the team says that their model is perfectly situated to help physicians better target and irradiate the tumors of cancer patients. While radiation therapy today is diffuse and can fill an entire hospital room, another co-author of the study, Robert Byer, co-director of theStanford Photonics Research Center, said in a statement that an accelerator-on-a-chip would be small enough to target just specific tumors.
“In this paper we begin to show how it might be possible to deliver electron beam radiation directly to a tumor, leaving healthy tissue unaffected,” said Byer.
Sapra tells Inverse that their chip is in no way trying to replace traditional accelerators like CERN’s Large Hadron Collider, but that they hope this alternative approach could help democratize access to such technology.
“The scalability of DLAs is important for their ability to democratize and make accelerators more widespread,” says Sapra. “These accelerators are made using the same semi-conductor nanofabrication techniques which allow consumer electronics to be made relatively cheap. Similarly, we are enthusiastic to work towards a table-top, low-cost particle accelerator for doctors, industry, and researchers.”
But to achieve these goals, the team will first need to accelerate their electrons at 94 percent (or 1 million electron volts) the speed of light, and they’re not quite there yet. Their model currently provides only a single stage of acceleration, during which the electrons gained .915 kilo-electron volts. In order to reach their 1 million electron volts threshold, the electrons will need to go through 1,000 acceleration stages on that single chip.
Abstract:
Intraoperative diagnosis is essential for providing safe and effective care during cancer surgery. The existing workflow for intraoperative diagnosis based on hematoxylin and eosin staining of processed tissue is time, resource and labor intensive. Moreover, interpretation of intraoperative histologic images is dependent on a contracting, unevenly distributed, pathology workforce. In the present study, we report a parallel workflow that combines stimulated Raman histology (SRH)5–7, a label-free optical imaging method and deep convolutional neural networks (CNNs) to predict diagnosis at the bedside in near real-time in an automated fashion. Specifically, our CNNs, trained on over 2.5 million SRH images, predict brain tumor diagnosis in the operating room in under 150 s, an order of magnitude faster than conventional techniques (for example, 20–30 min). In a multicenter, prospective clinical trial (n = 278), we demonstrated that CNN-based diagnosis of SRH images was noninferior to pathologist-based interpretation of conventional histologic images (overall accuracy, 94.6% versus 93.9%). Our CNNs learned a hierarchy of recognizable histologic feature representations to classify the major histopathologic classes of brain tumors. In addition, we implemented a semantic segmentation method to identify tumor-infiltrated diagnostic regions within SRH images. These results demonstrate how intraoperative cancer diagnosis can be streamlined, creating a complementary pathway for tissue diagnosis that is independent of a traditional pathology laboratory.