Could quantum molecules form a new periodic table?
They're artificial atom building blocks that can change technology, if scientists could just wrangle them.
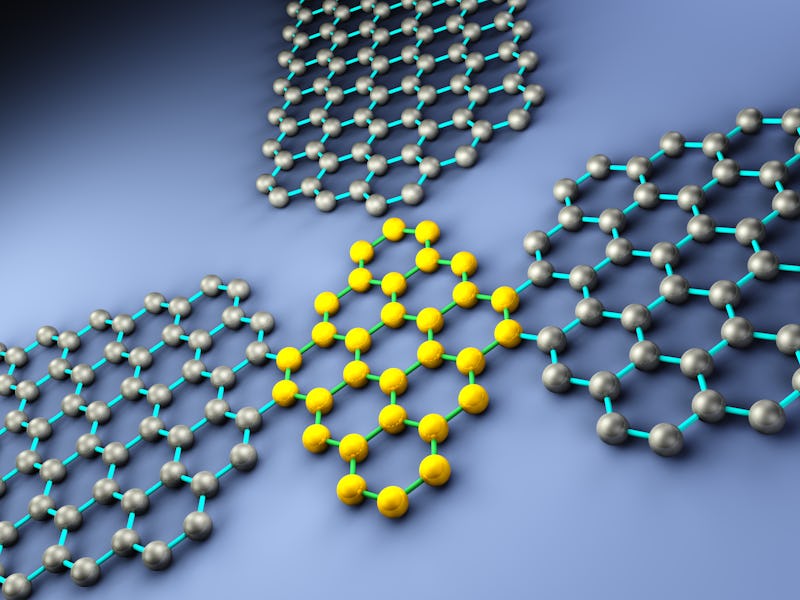
The periodic table of elements has shaped our understanding of the natural world for 150 years. Quantum chemistry has the potential to change one of the most famous scientific charts in history, in a big way.
The periodic table, which to date contains 118 natural and laboratory created elements, was famously stumbled upon in a dream by Russian chemist Dmitri Mendeleev in 1869 and describes the atomic properties of elements ranging from hydrogen (the simplest element) to the more exotic oganesson. And now, new research from the Hebrew University of Jerusalem suggests that a whole new class of elements might be joining the table: quantum dot “atoms.” But you probably shouldn’t hold your breath waiting for the new quantum table design to drop.
While the study’s lead author, Uri Banin, told the The Jeruselum Post that while the periodic table comparison is more of an analogy than a direct parallel, the research itself has the potential to enhance the development of new sensing and biological tagging technologies.
The research was published Monday in the journal Nature Communications and describes an approach to nanocrystal chemistry which enables researchers to fuse together colloidal quantum dot “atoms” to form new “molecules.” These quantum dots themselves are nanometer-sized crystal pieces that contain thousands of semiconductor atoms. While far from traditional atoms, the researchers write that these quantum atoms possess characteristics similar to “real” atoms, namely different atomic excitation levels.
Unlike their elemental counterparts, quantum dots are far less predictable than periodic elements. Based on their size, instead of material, these dots can change their optical and electronic properties, a trait that has actually been utilized to create next-generation color quality in TVs.
It’s not just sharper TVs, either. Quantum dots are worth the complication of nanotechnology, scientists feel, because of the wide array of uses. Quantum dots can be thought of as tiny semiconductor particles, meaning they have a wide range of electronic and optical properties ready to be exploited. Understanding quantum dots could lead to everything from better solar cells to sharper laptop cameras.
The authors write that scientists have gotten better and better at controlling these capricious quantum dots and that this control is essential in allowing them to even consider something like a quantum dot molecule. Just as atoms come together to create molecules with different unique characteristics, the team set out to discover how they could fuse quantum dots together to create an analogous result.
“If one considers [quantum dots] as artificial atom building blocks, how plentiful would be the selection of composition, properties, and functionalities of the corresponding artificial molecules?” the authors ask in the paper.
The research team used what is called a “colloidal synthesis,” a multi-step synthetic method capable of producing high numbers of artificial molecules. Using this approach structurally and electronically couple, or fuse, two of these quantum dots to form a quantum dot molecule. The researchers write that the quantum dot molecule could be observed exhibiting electronic coupling at room temperature — thanks to its visible redshift — and that its energy absorption response was also broadened as a result of the coupling.
Banin tells Inverse that these combined molecules even demonstrated benefits that quantum dots alone did not, including better bioimaging capabilities that would allow physicians to non-invasively view biological processes (e.g. quantifying metabolite and ion levels) in real-time.
“A main problem with some of the implications of quantum dots in devices is a blinking phenomenon, in which the quantum dots suddenly turns ‘off’ and don’t emit any color for a certain time,” says Banin. “In our synthesized molecule, we demonstrated that this ‘turn-off’ process is less pronounced. Another example would be the ability to synthesize a molecule that has a dual-color emission, which is not possible in quantum dots. This will be beneficial in bio-imaging, for example, where it might emit in a different color each time according to changes in the surroundings hence relevant for various sensing applications.”
And while the quantum dot molecules described in the study were made using the same quantum dots — and thus had very similar properties to the original dots — Banin says this wouldn’t always be the case.
“The difference between the quantum dot molecule and the quantum dots themselves is dependent on the chosen system,” says Banin. “In [our] paper, constituting the first example of such artificial molecules, a homo-dimer molecule was presented, which is the simplest artificial molecule model. This means that two similar quantum dots were used for creating the molecule. If there was no coupling between the quantum dots, looking at the emission spectrum of the molecule we should have seen a similar emission to that of the individual quantum dots….. In the future works - when designing different artificial molecules, with heterogeneous quantum dots, different properties will be manifested by the molecule, depending on the difference between the composing quantum dots.”
The authors write that they hope this research will provide a solid footing for future nanocrystal chemistry and that the unique properties of these quantum dots can be used to further advance sensing and quantum technologies.
Update: This article has been updated to include researcher responses.
Abstract:
Coupling of atoms is the basis of chemistry, yielding the beauty and richness of molecules. We utilize semiconductor nanocrystals as artificial atoms to form nanocrystal molecules that are structurally and electronically coupled. CdSe/CdS core/shell nanocrystals are linked to form dimers which are then fused via constrained oriented attachment. The possible nanocrystal facets in which such fusion takes place are analyzed with atomic resolution revealing the distribution of possible crystal fusion scenarios. Coherent coupling and wave-function hybridization are manifested by a redshift of the band gap, in agreement with quantum mechanical simulations. Single nanoparticle spectroscopy unravels the attributes of coupled nanocrystal dimers related to the unique combination of quantum mechanical tunneling and energy transfer mechanisms. This sets the stage for nanocrystal chemistry to yield a diverse selection of coupled nanocrystal molecules constructed from controlled core/shell nanocrystal building blocks. These are of direct relevance for numerous applications in displays, sensing, biological tagging and emerging quantum technologies.