Scientists examine the twisted dynamics of robotic muscles
Soft fibers are "the basic unit" of robotic muscle. Now scientists are figuring out they work.
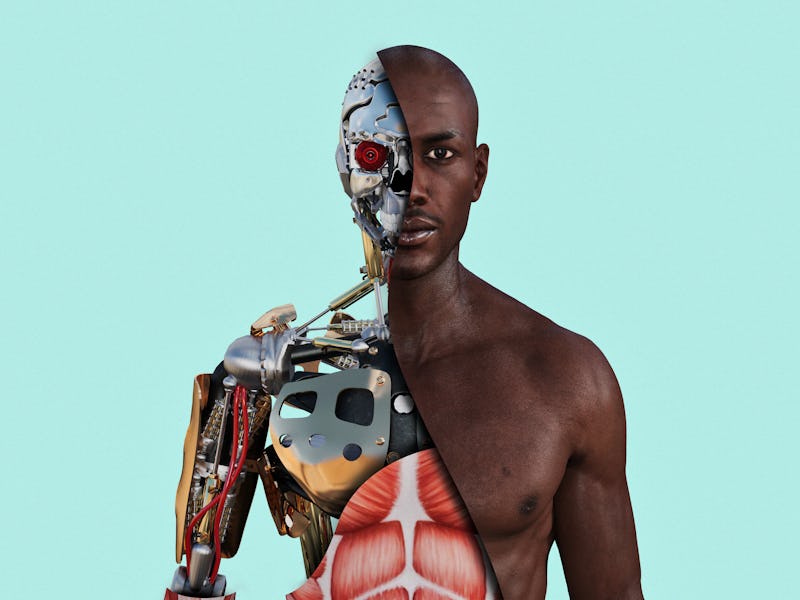
As robots become more and more lifelike, this begs the obvious question: how will they keep their robotic muscles in tip-top shape?
While they’re hitting robotic gyms just yet, researchers have discovered new information about how the different geometries and topologies (aka shapes and tensions) of a robotic muscle affect their stability and functionality.
Similar to a rubber band, artificial muscles are created using thin, soft filaments that are capable of creating energy and contracting just like “real” human muscles. This technology is already being implemented in robot prototypes, but in order to create better, next-generation muscles the researchers of a new study say it will be important to better understand the underlying mechanics of these “muscles.”
The study was published Wednesday in the journal Physical Review Letters and describes a systematic approach the researchers used in order to test the stability of these muscles under different forces to see how they might stretch, shear, bend, or twist. To imagine this in practice, think about twisting a rubber band. As you more tightly twist it the band will, seemingly at random, contort itself into a non-linear coil or twist. The researchers sought to discover how an artificial muscle might similarly react and whether those different forms would affect the muscle’s overall stability.
Better understanding those underlying design principles is an important yet overlooked aspect of this area of research, coauthor and Harvard Professor of Applied Mathematics, Organismic and Evolutionary Biology, and of Physics, L. Mahadevan, said in a statement.
“Thin soft filaments that can easily stretch, bend, twist or shear are capable of extreme deformations that lead to knot-like, braid-like or loop-like structures that can store or release energy easily,” said Mahadevan. “But how the topology, geometry and mechanics of these slender fibers come together during this process was not completely clear. Our study explains the theoretical principles underlying these shape transformations, and sheds light on the underlying design principles.”
Researchers tested different forms of twists in a computational model to determine how to best coil a robotic muscle in order to create stability and function.
In their experiments, the researchers found that artificial muscles that were pre-stretched before having other forces applied to them were more likely to take on an organized coiled shape versus a more kinked loop. They determined that these coils resulted in more muscle stability and were able to better conserve and expend energy when the tension was released and the coils straightened.
Nicholas Charles, a PhD student in Applied Mathematics and first author of the paper, said in a statement that this combined geometric, topological and mechanical understanding of these muscles can help create guidelines to build better artificial muscles in the future.
“Soft fibers are the basic unit of a muscle and could be used in everything from robotics to smart textiles that can respond to stimuli such as heat or humidity,” said Charles. “This research gives us a simple way to predict how soft filaments will respond to twisting and stretching.”
While these flexible fibers are generally imagined as muscles, Mahadevan also said that this basic understanding of twists, tension and coils could be applicable for other research as well including better understanding the dynamics of magnetic field lines, or how magnetic fields influence the world around them.
Abstract:
Soft elastic filaments that can be stretched, bent, and twisted exhibit a range of topologically and geometrically complex morphologies. Recently, a number of experiments have shown how to use these building blocks to create filament-based artificial muscles that use the conversion of writhe to extension or contraction, exposing the connection between topology, geometry, and mechanics. Here, we combine numerical simulations of soft elastic filaments that account for geometric nonlinearities and self-contact to map out the basic structures underlying artificial muscle fibers in a phase diagram that is a function of the extension and twist density. We then use ideas from computational topology to track the interconversion of link, twist, and writhe in these geometrically complex physical structures to explain the physical principles underlying artificial muscle fibers and provide guidelines for their design.