Big differences between expert and novice brains reveal a better way to learn
"That process causes big changes in the brain."
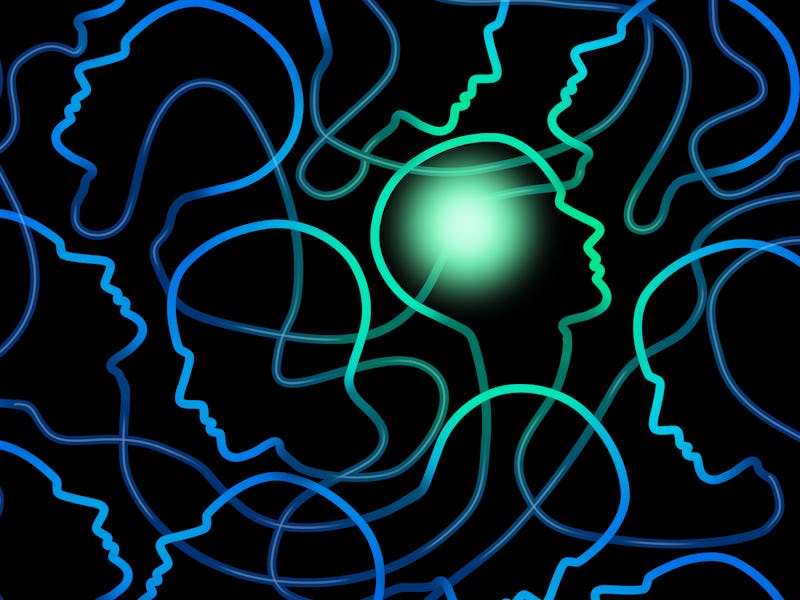
Even if you’re using advanced practice techniques, learning something new can be a struggle. These struggles are beneficial: With each mistake, the brain becomes a finely tuned neural machine. New research suggests that by the time expert status is achieved, the brain works differently than it did before.
Analysis of mouse brains by scientists at Cold Spring Harbor Laboratory in New York reveals the intricate ways that the brain changes during learning. Though this study was done in mice, senior study author and neuroscientist Anne Churchland tells Inverse that these findings indicate that human brains also change during the pursuit of mastery. Furthermore, the cells in the brain learn to fire in unique and specific ways throughout adulthood.
“We saw really big changes in the brains of adult mice that suggest even adult mice as capable of figuring out a totally brand new environment,” Churchland tells Inverse “That process causes big changes in the brain.”
In turn, Churchland is hopeful that these results can lead to new methods to improve learning. This research was published Monday in Neuron.
Green are excitatory mouse brain neurons and red are inhibitory neurons. These neurons become faster and respond more immediately in expert brains.
The type of learning that Churchland and the team looked at in this study isn’t something like learning a new language or memorizing a series of facts. You could think of it more like life-skills learning: It’s about how to navigate a foreign environment when you’re in over your head.
Churchland explains that the human equivalent would be when you find yourself in a totally new, fish-out-water situation.
“Maybe if you’ve been spending your whole life in a city and then you go on a canoe trip in Northern Alaska,” she says. “You don’t know anything about how to put up tents or how to avoid bears. You’re just completely starting from ground zero and you have to just figure that environment out.”
To recreate that kind of chaos, the team put mice into a stimulating environment filled with new decision-making tasks for them to master. The mice received rewards once they figured out how to make the right decisions, like drinking from a water bottle following a series of audio and visual cues. Over the course of about months they analyzed the way neurons in their brains fired as they went from novices to experts.
The environment the mice were in mimics what it would be like to be in a completely foreign environment and have to learn new skills quickly, from scratch.
As the mice progressed, more and more neurons became involved in the learning process, and they also started to respond faster. But most importantly, they found that the neuron activity became more selective — the neurons became pickier about when they fired and when they didn’t.
Essentially, non-selective neurons will respond no matter how a mouse behaves, even if it makes the wrong choice and doesn’t receive a reward. The novice brain is filled with these non-selective patterns of neuron response, says Churchland.
By comparison, the expert brain is filled with very specific patterns of response. These selective neurons only respond when the mouse makes one choice and not another. As the mouse learns that one way of doing things is better than another (and becomes an expert in that activity), that pattern becomes stronger.
Eventually, when expertise is reached, the pattern is so clear that Churchland says they could see the mouse’s brain preparing for that expert decision before they even execute it. By comparison, when a mouse is merely a novice, they couldn’t tell by neuron activity what the mouse would do until the moment that it made a choice.
A “better way” to learn
Churchland’s work provides a deep look into what happens in the brain when a mouse becomes an expert at something, which isn’t a perfect parallel to humans. But in general, they suggest that expertise is underpinned by extremely strong and predictable patterns of brain activity that are learned over time.
"That process causes big changes in the brain."
Understanding that process, says Churchland, suggests there might be ways to actually help human adults learn things faster. Her previous research, together with this paper, points to one way to do this. Animals tend to learn new things more efficiently when they experience new information in a variety of ways: through visual and auditory stimuli, she says. That principle could hold true for humans too:
“I think the take-home to apply to humans is that if you ever have the chance to learn in a multi-sensory context, it’s probably a much better way to learn,” she says. “You’ll probably learn much faster.”
The results also suggest you should give your adult brain some credit: It’s capable of undergoing some big changes that can help you hone expertise, and giving it plenty of different types of information to work with could help speed the process along.
Abstract: Inhibitory neurons, which play a critical role in decision-making models, are often simplified as a single pool of non-selective neurons lacking connection specificity. This assumption is supported by observations in primary visual cortex: inhibitory neurons are broadly tuned in vivo, and show non-specific connectivity in slice. Selectivity of excitatory and inhibitory neurons within decision circuits, and hence the validity of decision-making models, is unknown. We simultaneously measured excitatory and inhibitory neurons in posterior parietal cortex of mice judging multisensory stimuli. Surprisingly, excitatory and inhibitory neurons were equally selective for the animal’s choice, both at the single cell and population level. Further, both cell types exhibited similar changes in selectivity and temporal dynamics during learning, paralleling behavioral improvements. These observations, combined with modeling, argue against circuit architectures assuming non-selective inhibitory neurons. Instead, they argue for selective subnetworks of inhibitory and excitatory neurons that are shaped by experience to support expert decision-making.