A Vigorous Devourer of Greenhouse Gas Is Living Beneath Our Feet
"It just seems to be really, really, really efficient."
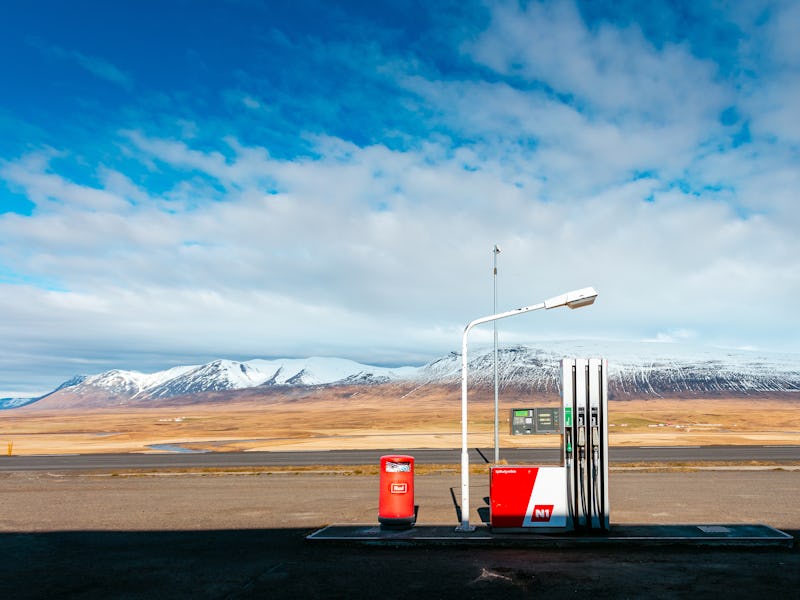
As the effects of greenhouse gases on climate change become increasingly apparent, scientists are leaving no stone (or field) unturned in their search for solutions. On Monday in PNAS, they reported that a small but mighty ally in the fight against climate change may be hiding right beneath our feet — and we’ve been destroying it without even realizing.
In the paper, European researchers report that they’ve isolated and grown a species of soil bacteria that lives on methane, the potent greenhouse gas that is second in importance only to the almighty carbon dioxide.
That bacterium is Methylocapsa gorgona, which lives in soil all over the Earth. Because it can live on methane present in extremely low concentrations, the feisty microbe can pull the gas out of the atmosphere and consume it, even when it’s not near a major source.
“We were lucky to get this atmospheric methane oxidizer in pure culture in the laboratory,” Mette Marianne Svenning, Ph.D., a professor of arctic and marine biology at The Arctic University of Norway and the corresponding author on the new paper, tells Inverse. Svenning’s group is the first to successfully isolate the bacterium responsible for methane storage in soil.
“Several research groups have hunted for these since it has been known that soil can take up methane from the atmosphere,” she says.
Bacteria in rice paddies produce methane, but recently scientists have started culturing bacteria that consume it. Could M. gorgona join the arsenal of greenhouse gas-eating bacteria?
Sky and Soil
The team is hopeful that they will eventually be able to harness M. gorgona to eat more and more methane out of the sky, but for the time being they’re focusing on its role in the ground. The soil acts as an important methane sink, partially because of bacteria like M. gorgona, but human activities are making soil less capable of storing methane.
Peter Dunfield, Ph.D. is a professor of microbiology at the University of Calgary who wasn’t involved in the new paper but has been studying methane-eating bacteria (methanotrophs) for many years. He tells Inverse that this new research is an exciting development in the area of soil bacteria.
“It’s always been a mystery: How do these really high-affinity methanotrophs manage to live on that tiny little amount in the atmosphere?” he says. Now we have a better idea.
Dunfield, whose work is cited multiple times in the new paper, says that several other strains of methanotrophs are already used in high-methane environments like rice paddies, where they’re used as methane filters to help reduce the amount released into the atmosphere. But whereas those strains can only live on very high concentrations of methane, M. gorgona can live on extremely low concentrations — as low as 1.86 parts per million. This tells scientists that the bacterium is highly efficient at using methane as a food source.
But perhaps the most interesting thing about M. gorgona is how similar it is to these other methanotrophs, despite its massive efficiency advantage, says Dunfield.
“The fascinating thing to me about the paper is we kind of expected them to be very different because of that — and they’re not. They’re actually pretty similar,” he says. “There’s nothing spectacular that jumped out and said, ‘Ah, this is a special organism, and it oxidizes methane in a completely different way.’ That’s not the case. It just seems to be really, really, really efficient.”
This deeper understanding of M. gorgona helps explain why soil is such an efficient methane sink. It also helps explain why soil isn’t soaking up more methane.
“Anthropogenic activity — agriculture, tillage, all kinds of things seem to destroy it — so certainly understanding the exact organisms that perform the process could be worthwhile in understanding how not to destroy them,” says Dunfield.
Therefore, preserving the methanotroph in soils could help slow the rate at which methane accumulates in Earth’s atmosphere and warms the planet.
As far as innovative approaches to scrubbing methane from the atmosphere, the researchers behind the paper are hesitant to say that their research unlocks creative new solutions to climate change — at least not yet.
Nevertheless, M. gorgona’s ability to consume methane from the atmosphere suggests that it could be supercharged to suck more out, slowing the rate of global warming. Svenning says it may be too early to do that, but the methane filters of the type Dunfield mentioned give her hope that scientists will imagine ways to incorporate the newly discovered bacterium into creative solutions.
“The application is somehow difficult to see now, but new knowledge can lead to applications that we don’t see at the moment,” she says. “The way we cultivate the bacterium on floating filters is innovative and can hopefully contribute a wider approach in environmental microbiology and new isolates.”
Abstract:
The global atmospheric level of methane (CH4), the second most important greenhouse gas, is currently increasing by ∼10 million tons per year. Microbial oxidation in unsaturated soils is the only known biological process that removes CH4 from the atmosphere, but so far, bacteria that can grow on atmospheric CH4 have eluded all cultivation efforts. In this study, we have isolated a pure culture of a bacterium, strain MG08 that grows on air at atmospheric concentrations of CH4 [1.86 parts per million volume (p.p.m.v.)]. This organism, named Methylocapsa gorgona, is globally distributed in soils and closely related to uncultured members of the upland soil cluster α. CH4 oxidation experiments and 13C-single cell isotope analyses demonstrated that it oxidizes atmospheric CH4 aerobically and assimilates carbon from both CH4 and CO2. Its estimated specific affinity for CH4 (a0s) is the highest for any cultivated methanotroph. However, growth on ambient air was also confirmed for Methylocapsa acidiphila and Methylocapsa aurea, close relatives with a lower specific affinity for CH4, suggesting that the ability to utilize atmospheric CH4 for growth is more widespread than previously believed. The closed genome of M. gorgona MG08 encodes a single particulate methane monooxygenase, the serine cycle for assimilation of carbon from CH4 and CO2, and CO2 fixation via the recently postulated reductive glycine pathway. It also fixes dinitrogen and expresses the genes for a high-affinity hydrogenase and carbon monoxide dehydrogenase, suggesting that atmospheric CH4 oxidizers harvest additional energy from oxidation of the atmospheric trace gases carbon monoxide (0.2 p.p.m.v.) and hydrogen (0.5 p.p.m.v.).