Scientists May Use Lasers to Gauge if "Super-Earths" are Fit for Life
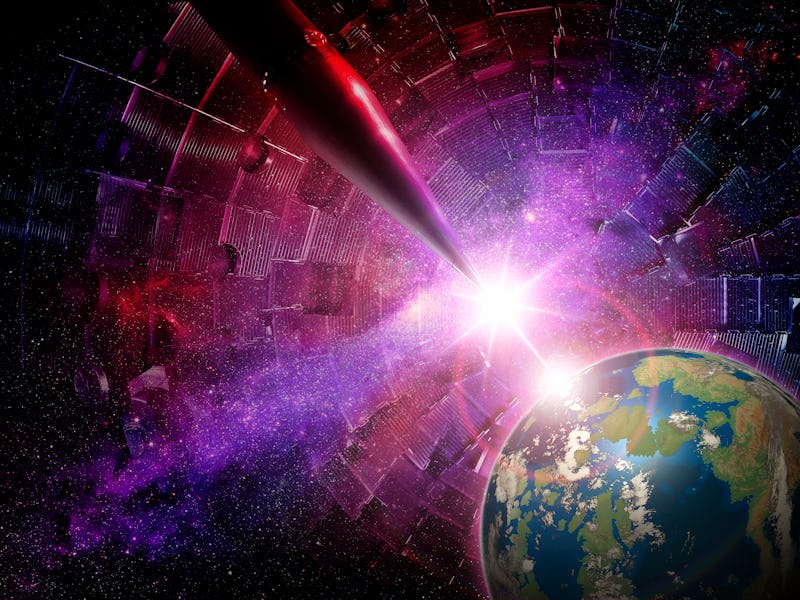
So-called “super-Earths” are the exoplanet du jour, probably because they remind us so much of home. These worlds — which have a mass higher than Earth’s but less than Neptune’s — are thought to be fairly common outside our solar system. Now, scientists using lasers to test iron samples in an experiment that could set up how we one day will gauge whether super-Earths are fit for life.
In a new study published Monday in Nature Astronomy, physicist Raymond F. Smith and his colleagues describe why they used high-powered lasers to perform a compression experiment using the common element iron.
“This paper describes a new generation of high-power laser experiments, which uses quasi-isentropic ramp-compression techniques to provide the first absolute equation of state measurements of [iron] at the extreme pressure and density conditions found within Super-Earth cores,” Smith writes in a statement shared with Inverse. “Such shock-free dynamic compression is uniquely suited for compressing matter with minimal heating to TPa pressures.” For context, one Tpa, or terapascal, is equivalent to 10 million atmospheres.
Smith and his team performed a ramp compression experiment on an iron sample at the Lawrence Livermore National Laboratory in Livermore, California. In order to compress iron to 1.4 TPa, the group used copper foil glued onto an iron sample to simulate an all-iron exoplanet. Then, they fired away with lasers.
Scoping out an exoplanetary core.
“The energy from 176 laser beams was converted into an x-ray drive,” Smith explains. “Our pressures are four times higher pressure than previous static results and represents core conditions found with a three to four Earth-mass planet.
Smith says their results “provide the first experimentally based mass-radius relationship for a hypothetical pure-iron planet at super-Earth core conditions,” which can be used to determine the “plausible compositional space for large, rocky exoplanets.”
Iron is a sensible sample to use because there’s a ton of it throughout the universe. Extremely massive stars can leave behind iron as a result of nuclear fusion, which is how they create energy. By understanding the cores of certain super-Earths, scientists can create better models to determine the surface conditions of these planets. This can be helpful in order to determine the habitability of extrasolar worlds.
In short, this work is a stepping stone for future astronomers and physicists in their exoplanetary research.
Speaking of the future, it’s looking pretty solid for exoplanet fans. On Monday, NASA will launch its newest exoplanet hunter, TESS, on board a SpaceX Falcon 9 rocket. Using state of the art cameras with 16.4-megapixels, TESS will be able to observe distant worlds in unprecedented detail.
Abstract:
The recent discovery of thousands of planets outside our Solar System raises fundamental questions about the variety of planetary types and their corresponding interior structures and dynamics. To better understand these objects, there is a strong need to constrain material properties at the extreme pressures found within planetary interiors. Here we used high-powered lasers at the National Ignition Facility to ramp compress iron over nanosecond timescales to 1.4 TPa (14 million atmospheres) — a pressure four times higher than for previous static compression data. A Lagrangian sound-speed analysis was used to determine pressure, density and sound speed along a continuous isentropic compression path. Our peak pressures are comparable to those predicted at the centre of a terrestrial-type exoplanet of three to four Earth masses, representing the first absolute equation of state measurements for iron at such conditions. These results provide an experiment-based mass–radius relationship for a hypothetical pure iron planet that can be used to evaluate plausible compositional space for large, rocky exoplanets.