The Secret to Quantum Computing Might Be in an Old Paper from 1998
"This could have been thought of 20 years ago."
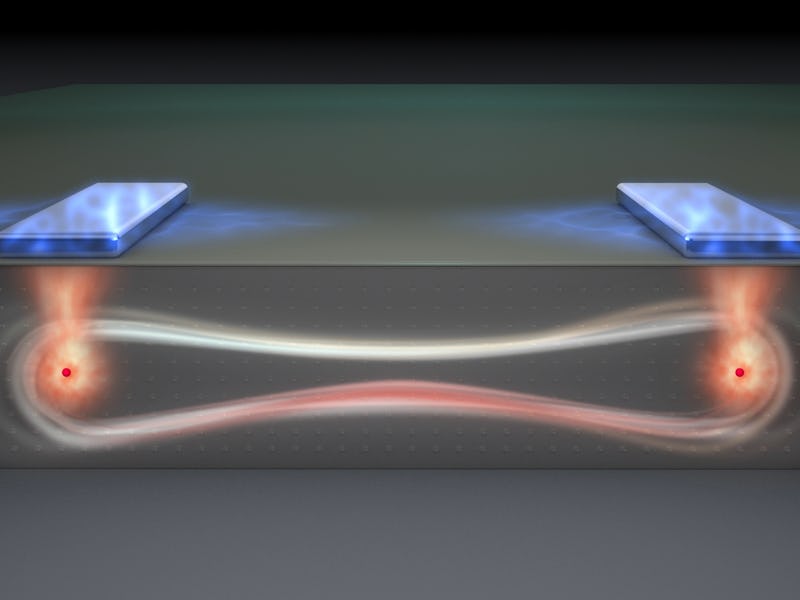
We already know how to build quantum computers, at least theoretically speaking. It’s simple enough: Just take the atom-sized objects that are the individual units of quantum information, or “qubits,” and place a million of them on a quantum version of the classical silicon computer chip. Do that, and you’ve got the most powerful computer ever created.
Theoretically, it’s easy. Practically, it’s an engineering nightmare, considering each qubit has to be only about 10 nanometers — that’s 10 billionths of a meter, or about 50 atoms wide — apart from one another in order to interact.
“It means that all the components, all the interconnects, all the readout transistors need to be on that scale,” Andrea Morello, a leading quantum computer researcher, tells Inverse. “So the density becomes really high. It’s not impossible, but it’s challenging.”
Morello and his colleagues at the University of New South Wales in Sydney, Australia, may have found a way to sidestep that challenge, as they detail in an article published Wednesday in Nature Communications. If they are right, they have brought us a big step closer to the quantum computing revolution, which would let us perform unimaginably complicated calculations that would flummox classical computers.
Andrea Morello (left) with fellow researcher Guilherme Tosi
The researchers seized upon an idea that’s been staring quantum computing researchers in the face since fellow New South Wales researcher Bruce Kane came up with his revolutionary design for such a computer in a 1998 paper.
“This could have been thought of 20 years ago,” says Morello. “It just hasn’t,” he adds with a laugh.
The idea is that instead of using just an atom’s electron or just its nucleus as the qubit, using the combination of both creates a qubit that’s also an electric dipole, meaning positive and negative charges are separated. The fine-grained technical details don’t matter too much, but here’s the key point: These new qubits can still interact with each other and engage in all necessary quantum weirdness at distances of as much as 200 nanometers. Compared with 10 to 20 nanometers, that’s a ton of breathing room.
“You’ve got way more space to put all the infrastructure around,” he says. “For the actual large-scale quantum computers we want to build in the next decade or so, this is going to make life much easier.”
But the extra space doesn’t mean any huge sacrifice in terms of potential quantum computing power, as it would still be possible to fit a million of these so-called flip-flop qubits on a single quantum computing chip.
It’s such a straightforward idea — at least by the standards of quantum computing — that it’s remarkable it’s taken researchers this long to notice the possibility. Morello chalks that up to the nature of serendipity, a reminder that there’s always a human element to any research. He and his colleagues made this connection, but it’s long been waiting to be made.
The idea of a magnetic dipole was lurking in one of the original figures for Bruce Kane's 1998 paper.
“When you look at the old papers, some of the embryos of this idea were there,” says Morello. “But no one realized there was an electric dipole being created when you do that. It’s even in the images. If you look at the 1998 Kane paper with what we know now, you look at that figure, and you think, ‘Of course, there’s an electric dipole there.’ But it’s not discussed in the paper, it’s not discussed in any paper for 19 years after that.”
Morello says his team are doing experiments on this new design, and there’s preliminary evidence that it works, though he can’t talk too much about that before they are ready to publish results.
If it works, this new design represents the ideal middle ground between the terribly crammed together conditions of current qubits on a silicon chip and the much larger qubits found in superconducting quantum computer. This represents an entire alternative stream of research, one where it’s much simpler to make progress, but their potential is limited compared with what can happen on a silicon chip.
“They are the ones that are arguably ahead of the pack at the moment,” Morello says of superconducting qubits. “And part of the reason is because they are so big. They are so easy to fabricate. But you’re not going to fit a million of them on a square millimeter. In fact you will barely fit one on a square millimeter.”
Getting a million qubits on a silicon quantum chip is still a long way off, considering the current record is only two. Morellos says the near-term goal is to get to 10 qubits in five years. He adds a million could be a possibility in 20 years, but he stresses this is only a guess.
In the meantime, Morello says this new research has him hugely more optimistic about making real headway in quantum computing research.
“I’ve been working in this specific field of silicon quantum computing for 10 years and I love it, I’ve been successful in it, but there comes a point where you think, okay, how far can I push it?” he says. “And, two years ago, I thought I could see the next couple of qubits coming together. Couldn’t really see the million qubits. And now I can.”