Nuclear fusion breakthrough: A physicist answers three vital questions
We asked a physicist about the future of nuclear fusion.
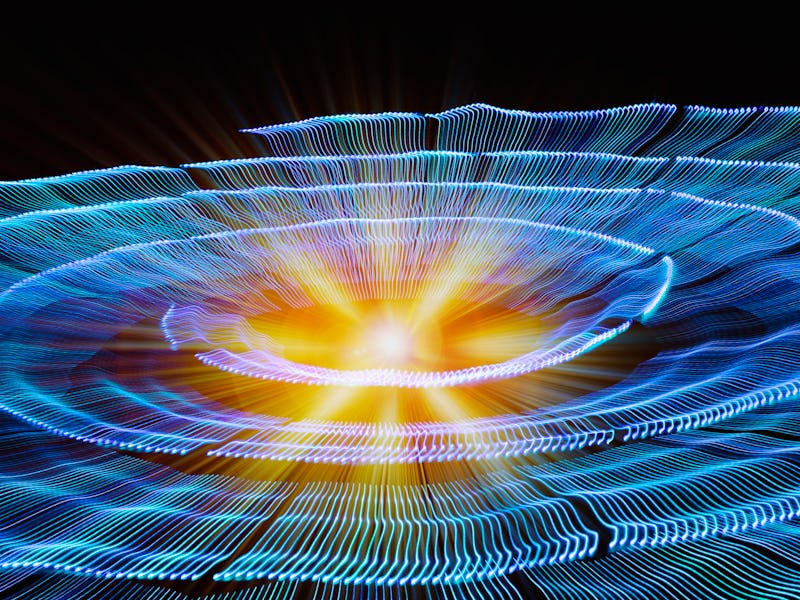
On December 5, scientists at the Lawrence Livermore National Laboratory took the first step toward harnessing a new abundant, clean form of energy. For the first time, they harnessed extra power from nuclear fusion, a reaction in which hydrogen is heated up to extremely high temperatures and converted into helium — the process that keeps stars like the Sun glowing above us.
To replicate this phenomenon on Earth, the team blasted 192 laser beams at an eraser-sized gold cylinder and triggered an implosion that released 3.15 MJ of energy. Their successful experiment marked the first time that researchers have been able to create excess energy through nuclear fusion, or, a simpler way to look at it: get more juice than they put in.
But hold your horses: The lab triumph is just a small step toward nuclear fusion power plants. It took a humongous amount of energy (300 MJ) to send 2 MJ out of the laser and onto the fuel pellet containing the hydrogen isotopes deuterium and tritium.
At the National Ignition Facility, 192 laser beams blasted a pencil eraser-sized container with hydrogen isotopes inside.
And the experiment relied on decades-old technology. Now, it’s up to researchers to recreate this process with newer devices and scale things up. To jumpstart this investigative work, the White House aims to have a plant up and running within the coming decade. It isn’t clear, though, whether this lofty goal will succeed.
To parse through what the future of fusion holds, Inverse spoke with Stephanie Hansen, a physicist and senior scientist at Sandia National Labs who studies laser-powered fusion.
What does this breakthrough mean for the future of fusion energy?
The scientists at LLNL’s National Ignition Facility showed that we can heat and compress a little bit of material to temperatures and densities similar to those at the center of our Sun to create initial fusion reactions, Hansen says. Most importantly, the team was able to hold that material together for long enough to recapture and use that initial fusion energy to reach even more extreme temperatures (over 5 million degrees Fahrenheit) and burn more fusion fuel.
Past attempts have fizzled out: In the previous record set by NIF, scientists managed to produce 70 percent of the energy they put in, so they ended up with a net loss.
“This experiment demonstrated that we understand the physics of material and radiation at extreme conditions well enough to create and control an igniting plasma, which means we will have more confidence in future fusion target designs and innovations that could bring fusion energy to the grid,” she says.
What more work needs to be done to scale up laser-based fusion?
NIF’s conversion from grid-sourced energy to laser energy is inefficient, but laser tech has come a long way since the NIF device was planned in the 1990s. For one, more efficient lasers have since been developed, explains Hansen. Now, scientists can work on integrating newer tech with advanced laser-blasting methods.
There’s also the supply issue of hydrogen isotopes, as reported by Chemistry World. While there’s lots of deuterium on Earth, it produces a relatively low amount of energy. And tritium doesn’t appear naturally, so it needs to be created with the help of lithium. But lithium is already sought out in large quantities for batteries, which could create some sourcing competition.
The laser beams cause the fuel capsule to implode and kick-off nuclear fusion.
What type of technology could power fusion plants?
The tokamak design is being considered for future nuclear fusion plants.
In addition to blasting hydrogen fuel with lasers, scientists are also working on devices that use magnetic fields to trap hydrogen isotopes so that they heat up to the temperatures required for fusion to take place (which is 20 times hotter than the Sun’s core). These include the tokamak, a metal vacuum chamber invented by Soviet scientists in the 1960s, that has attracted mainstream hype in recent years.
“Magnetic confinement fusion has also made enormous strides in the last few years and holds significant promise as a continuous (rather than pulsed) fusion energy source,” Hansen says. “Although plasma densities, sizes, and time scales are vastly different between the two fusion methods, there are a lot of common physics and engineering challenges and many members of the two communities work closely together.”
Hansen thinks the exciting result from NIF could even spur innovative new ways to create fusion energy. In fact, studies have already looked into combining lasers and magnets: Scientists at NIF have applied a magnetic field to the fuel-containing capsule and found that it could increase the fusion energy threefold.
The precise formula to a successful fusion power plant remains elusive — but scientists could get there someday.