Why the Max Planck Institute Wanted to Cook Up Plasma in its Fusion Reactor
One million degrees is all it takes.
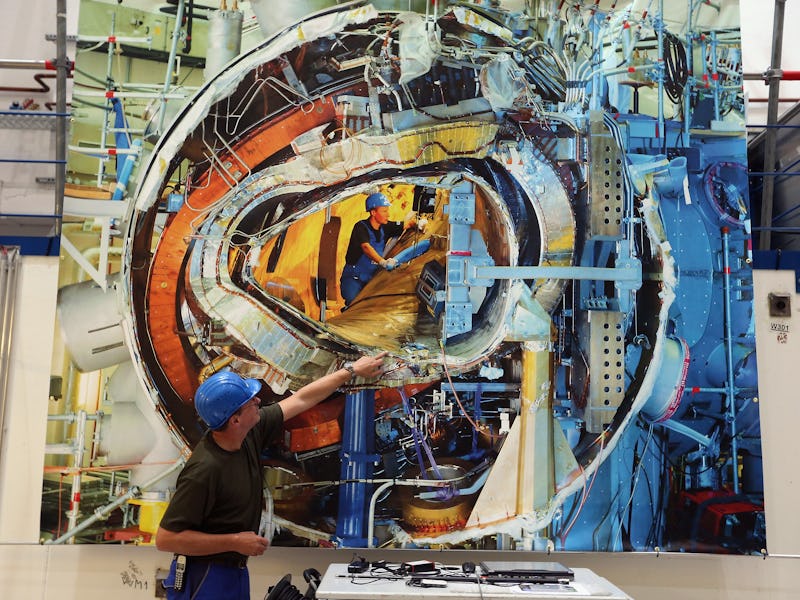
There’s nothing quite like a fusion reactor to generate excitement. After nine years of construction and €1 billion, scientists at the Max Planck Institute of Plasma Physics fired up the first hot test of the Wendelstein 7-X fusion device on December 10, and generated a helium plasma that lasted for one tenth of a second and reached a million degrees Celsius. But don’t get too hyped just yet. This was just a step toward preparing the device for its true purpose: studying nuclear fusion with hydrogen gas.
Okay, now you’re pumped.
Fusion has long been the golden calf of nuclear energy research, showing up nuclear fission in all categories except feasibility. Fusion produces a colossal amount of energy — it is, after all, the same process that powers the sun. But its very power makes it a pain in the ass to deal with. Every fusion reactor built so far consumed more power than it produced. The record for fusion power was set in 1997: 16 megawatts produced with an input power of 24 megawatts. But if someone manages to turn that equation around … Can you say cheap, carbon-free energy?
Unlike its less sophisticated cousin, fusion produces no radioactive waste. The hydrogen supply cycle is less problematic than the uranium supply cycle. To be fair, the most common sources of hydrogen today are coal and natural gas, but hydrogen could instead be produced by electrolysis.
Fission and fusion are alike in two respects. Both exploit the conversion of atoms of one element to atoms of another element, and both were first used as weapons. Fat Man and Little Boy, the fission bombs dropped on Hiroshima and Nagasaki in 1945, gave way by 1952 to fusion devices like Ivy Mike. (Although Ivy Mike was not built as a bomb, it was soon followed by thermonuclear warheads many megatons in yield all deliverable by intercontinental missile.)
The fusion bomb was known as an H-bomb for a reason: The unprecedented release of energy came from the fusion of hydrogen atoms. Fusion researchers seek to harness this effect for civilian power generation. Turns out this is a challenge. Hydrogen fusion at the Earth’s surface would require temperatures in excess of one million degrees Celsius. At these temperatures, hydrogen and helium become a plasma, the fourth form of matter.
But what the hell is a plasma, anyway?
In short, a plasma is an ionized gas. In a plasma, all molecular bonds dissolve and electrons leave their host atoms. Plasmas are highly conductive because they have a high charge carrier density, i.e. the electrons and ions are free to move independent of one another in response to an electric field.
Although this all sounds exotic, plasmas make regular appearances in our lives. The light from lightning bolts and neon signs comes from electrons recombining with ions and sinking to lower quantum states, a process known as spontaneous emission. Some flames are hot enough to ionize exhaust gasses, and plasma torches, plasma screens and arc welders all utilize plasmas.
The Wendelstein 7-X team produced a million degree helium plasma on December 10.
But all those have nothing on the plasma in a fusion reactor. At one million degrees Celsius, the atoms in the fusion soup are extremely energetic. If they’re not contained, they’ll jet off, damage the apparatus, and fail to fuse with one another. Without containment, you’d probably never reach one million degrees in the first place.
Containment is the major challenge in fusion research. The plasma must be kept in a confined space and must not touch the walls of the fusion vessel. Needless to say, the vessel must be kept at high vacuum. Wendelstein 7-X uses 65 vacuum pumps to hold the pressure at 0.000000001 millibars. (That’s 0.000001 Pascals for you SI lovers.) The only realistic means by which to confine an ionized gas at hellish temperatures is to hold it in a magnetic field. And this is where things get really tricky.
For years, the most popular fusion reactor design was the tokamak. In years before super-computers played chess, trashed humans at Jeopardy, and folded proteins, scientists came up with clever ways to produce the correctly shaped magnetic field. In a tokamak, an electric current running through the plasma pairs with external electromagnets to create the necessary magnetic field.
Not so in Wendelstein 7-X. Here, the containment field comes entirely from external superconducting electromagnets. The research team used a supercomputer to optimize the shape of these magnets and eliminate the need of a plasma current. This style of fusion reactor is known as a stellarator.
So far, no one’s built a fusion reactor that generates more energy than it consumes. Even Wendelstein 7-X, the largest stellarator-type reactor in the world, was built for research purposes, not to generate energy. But if you want to invest your hopes in a fusion project, Wendelstein 7-X is a good place to start. Make sure you keep an eye on ITER, too, set to be the world’s largest tokamak.