Neuralink: "Classic" 2003 Paper Is the Foundation for Elon Musk's Device
"We're, in the greatest sense, building on the shoulders of giants."
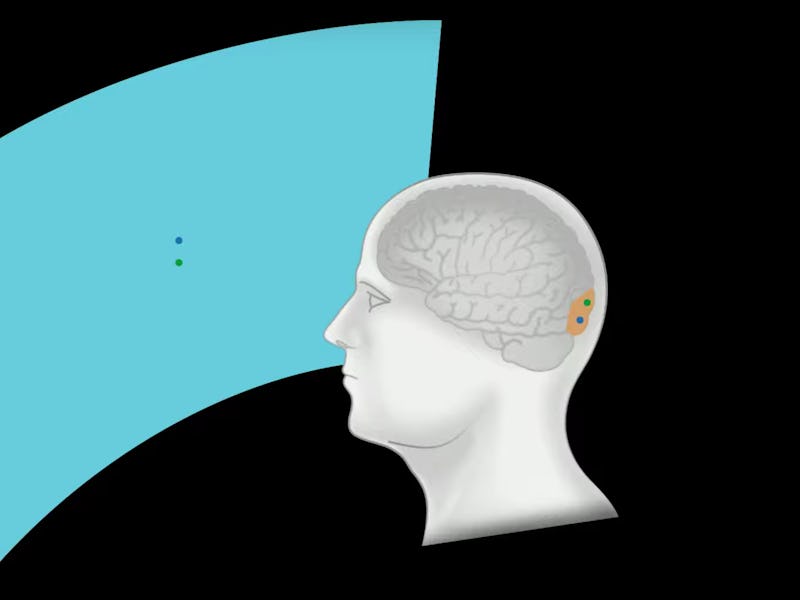
After over two years of speculation about Elon Musk’s much-hyped brain-computer interface, Neuralink, details of its development went public on Tuesday, with credit being given to a study that was published 16 years ago.
Musk and Neuralink president Max Hodak revealed that their implanted device could be ready for clinical trials as early as the end of 2020. They didn’t take all the credit, though: In his remarks, Hodak credited what he described as a classic paper in the field, published in PLOS One in 2003.
"We’re, in the greatest sense, building on the shoulders of giants."
The paper, written by a team led by Miguel A. L. Nicolelis, Ph.D., a celebrated specialist in brain-machine interfaces (BMIs) at Duke University, was the first to show how macaque monkeys could use their brains to make robotic arms reach and grasp.
“We’re, in the greatest sense, building on the shoulders of giants,” said Hodak, who previously did research at the Nicolelis lab at Duke.
N1 sensor.
The big reveal about the inner workings of Neuralink is that it involves four tiny “N1” sensors. One would be implanted into the brain’s somatosensory cortex, and three would be placed in motor areas. Each of these sensors is threaded with more than a thousand electrodes to collect the brain activity data on which the interface relies.
In 2003, the Nicolelis paper drove home the importance of two big questions: Where should those electrodes go, and how many of them are necessary to make a BMI work?
How BMIs Work
To understand how crucial the 2003 paper is to researchers at Neuralink, it’s important to understand how BMIs function generally. At their simplest, they involve a brain hooked up to a “machine,” whether that be a robotic arm, an A.I., or even another brain. Connecting the two parts creates an open line of communication between them that allows the brain, in time, to learn how to control the machine directly. (Whether communication will ever go in the other direction is an open, and troubling, question.)
Neuralink's breakdown of a BMI. A "machine" is a catch-all term for a robotic limb, A.I., or even another brain.
But what language do they use to communicate? This is where the electrodes become crucial. To assess what’s going on in the brain, neuroscientists look at brain activity, which refers to the electrical fields created by single neurons that, in turn, add up to patterns of electrical activity across the whole brain. Those patterns aren’t always rhythmic, but when they are, they become what we loosely refer to as “brain waves.”
If you were to chart the electrical activity of a single neuron over time, you’d see “spikes” of activity — kind of like a pulse on a heart monitor. These spikes, scientists have determined, are the best and simplest language we’ve got to allow brains and machines to communicate.
The Contributions of the Nicolelis Team and That 2003 Paper
Among many things Nicolelis and his colleagues figured out in their study was how many neurons — and which ones — they had to monitor to allow a macaque to control a robotic arm using its mind.
The short answer is a lot, spread out over many areas.
“Our previous studies have suggested that, because of the distributed nature of motor planning in the brain, neuronal samples from multiple frontal and parietal cortical areas ought to be employed to operate such devices,” the team wrote. Previous work looked at small samples of neurons (eight to 30 cells) and large ones (hundreds to thousands); their study focused on samples containing up to 64 cells.
Since many brain regions are involved in motor movement, it makes sense to draw information from all of them. And the more neurons they monitor, the better the results.
Hodak pulled up these graphs from the Nicolelis paper in his presentation on Tuesday. "R" on the y-axis refers to the goodness of fit for the model.
In his talk, Hodak pulled up two graphs from the Nicolelis paper showing the importance of monitoring many neurons. The data from these neurons was used to develop a model that would predict, in real time, the arm movements of the monkeys.
“As you add neurons, the quality of the model improves,” he said.
The Paper’s Impact on Neuralink
The N1 chip developed by Neuralink contains so many electrodes so it can monitor many neurons at once, building on the findings of the Nicolelis team. As Musk and the Neuralink team write in their white paper published July 16, “development of BMI has been critically limited by the inability to record from large numbers of neurons.”
Neuralink’s N1 chips are interwoven with miniscule, thread-like probes to reach thousands of neurons without damaging the brain. “We demonstrate the rapid implantation of 96 polymer threads, each thread with 32 electrodes, in a (4 × 7) mm^2 area of brain for a total of 3,072 electrodes,” the team writes.
That’s a lot of electrodes to draw information from a lot of neurons. But if the goal, as Musk has stated previously, is to achieve “symbiosis” between our brains and A.I., then the more information we can use for communication, the better. “If you have tasks that are more complicated, you need more neurons,” said Hodak as he discussed the Nicolelis paper.
"There is no wearable that is going to get you spikes."
While non-implantable devices, like an electroencephalogram cap or deep brain stimulation device, can also monitor many neurons at once, their reading of neuronal “spikes” is muddled, explained Hodak. “There is no wearable that is going to get you spikes,” he said. “This is a physics constraint.”
The Nicolelis lab, which is still doing work on BMIs, succeeded in training its macaques to use only their neurons to manipulate the robotic arm. A PLOS One editor highlighted the research in 2013:
…the monkeys soon learned to perform the task using their neurons alone — a true brain-machine interface. For some of these tasks the monkeys controlled a real robotic arm, making it squeeze a spongy object. Finally the authors took the pole away and found that the monkeys didn’t even try to use their arm muscles.
Now, 16 years later, the goal of BMIs has come a long way from using monkey brains to control robot arms. Once the considerable tech issues are addressed, write Musk and the Neuralink team, “it is plausible to imagine that a patient with spinal cord injury could dexterously control a digital mouse and keyboard.” Combined with spinal stimulation techniques, they conclude, “in the future this approach could conceivably restore motor function.”