Melting Gold With Lasers May Hold the Key to Surviving the Surface of Venus
Scientists at Stanford think gold can do a lot more than line space helmets.
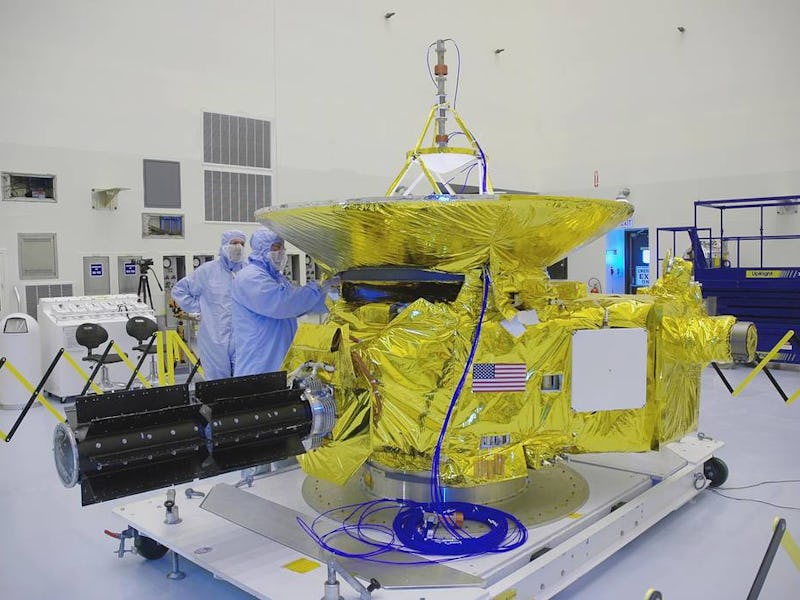
Gold has a lot of pretty cool industrial applications. NASA uses it to protect astronauts from cosmic radiation by lining the visors of their space helmets. Commercial electronics also use gold to plate contact points to prevent static and corrosion. But this may only be the beginning, says a group of researchers at Stanford who are battering gold with laser beams to understand the full extent of what the shiny metal can really do.
“We can now actually see what is happening on the atomic scale,” plasma physicist Siegfried Glenzer, director of the High-Energy-Density division at SLAC, tells Inverse. “That’s quite significant.”
Visualizing gold at the atomic scale required a number of technological breakthroughs, but that’s exactly what the National Accelerator Laboratory at the Stanford Linear Accelerator Center (SLAC) did recently, a feat which required developing a device that could watch what was happening to those individual atoms of gold at a timescale down near 100 femtoseconds (meaning 100 millionths of a billionth of a second).
“Previously,” Glenzer says, “we’ve had microscopes and we’ve had more and more improved tools, but this is really now the first time that we can see what atoms are doing, where the atoms are sitting, and then measuring how the atoms are moving around — where the atoms go as we excite the material.”
“Excite,” here, is a polite term for blasting gold with a pulsing ultraviolet beam until it reaches temperatures approaching those deep within Earth’s mantle, near its molten outer core. (The gold gets to about 3500K±500 K — or between about 4900 to 6700 degrees Fahrenheit. Gold’s melting point is 1948 degrees Fahrenheit.)
To actually capture what was happening with the gold as it melted, they basically had to manufacture a precise, “slow-motion camera” of sorts. What that means at the atomic scale is that the team at SLAC bounced a lot of incredibly fast-moving electrons off of the melting gold and then measured how those electrons behaved, a process called ultrafast electron diffraction (UED).
According to Glenzer, “You fire a laser beam on copper, you extract electrons, you catch those electrons in an electromagnetic field, and so on. Then you accelerate those.”
Glenzer’s group actually borrowed the free electron laser they needed from Stanford’s Linac Coherent Light Source (LCLS) facility.
“They had, like, a spare gun, and we were allowed to use that spare gun to build UED.”
A laser pulse melts solid gold (the dotted pattern) to a liquid (the ring pattern), as seen by SLAC’s ultrafast electron camera.
What We Can Learn by Melting Gold
So, how does gold melt when you torch it with an ultraviolet laser? What the group discovered was that the regions of gold with consistently spaced atoms in a well-organized crystalline latticework melted last, after all the more disorganized regions in between those homogenous areas melted first. It’s a little bit like ice cubes, if you’ve ever seen tiny bits of ice melt faster than large blocks of ice.
Understanding how these crystal lattice fault-lines in gold first fracture and then melt — and just generally react to energy bombardment — should help scientists and engineers design better protective gold materials for space travel, as well as for future fusion reactor experiments.
“In outer space, the environment is pretty harsh,” says Mianzhen Mo, a postdoctoral researcher at Stanford who has been focusing on the molecular geometry of “warm dense gold” and who played a pivotal role in this gold-melting research. “There’s super-energetic particles, ions, and protons. So, those particles are going to, like, hit your spacecraft — and those interactions, the bombardment, can actually change the material properties.”
Mo told Inverse he expects UED observational methods will also be used with other specialty materials designed for space and other extreme conditions. As an example, he mentioned silicon-carbide semiconductors that Stanford alumn Samira Motiwala has been investigating with NASA for use in a probe capable of surviving on the 864 degrees Fahrenheit surface of Venus.
“Previously,” Glenzer says, “they sent a device to Venus. I think it was a Russian probe. It survived for two minutes and then it was over.”
“And, actually, the conditions on Venus are still fairly okay compared to fusion reactors. Fusion reactors would be much more extreme.”
This is a gold-plated hohlraum mock-up from the National Ignition Facility, a laser-based inertial confinement fusion (ICF) experimental unit, at Lawrence Livermore National Laboratory in California.
High-tech materials are not the only area of scientific inquiry that might benefit from UED, however. Glenzer and Mo’s research, conducted by Stanford on behalf of the US Department of Energy Office of Science, and published last Friday in Science, could also expand into other fields where observing the ultrafast (and very tiny) atomic activities during molecular reactions might come in handy.
“People have begun using UED also to study chemical reactions, study reactions that are important in biology,” Glenzer says. “I think this is just the start.”