'Tides' Explores the Evidence of Earth's Rotational History
Follow Jonathan White's spiritual and scientific journey across the globe.
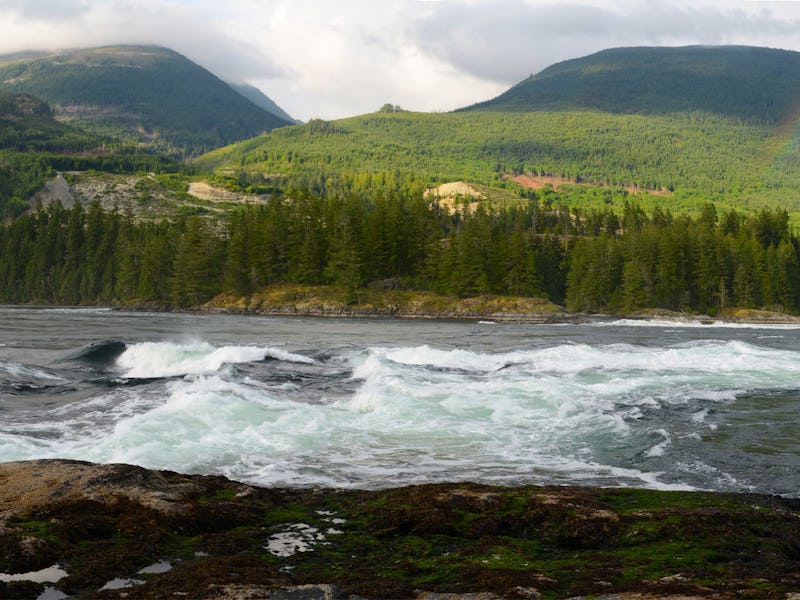
In his book, Tides: The Science and Spirit of the Ocean, Jonathan White embarks on a journey “across the globe to discover the largest, fastest, most dangerous and breathtaking tides in the world.”
In the following excerpt from Chapter 6, White travels to the Skookumchuck Narrows in British Columbia, where he dives into how humans have scientifically understood of the tides, while leaving room for the wonder that still remains in their movement. White writes on the history of time and how essentially nothing in life is constant, not even time, and that’s due in large part to the tides. It’s beautiful, imaginative, and rooted in philosophical questions laid out through history.
White writes of the physical evidence of Earth’s rotational history, too, which comes in the form of tidal rhythmites, “which are layers of soft stone formed on the seabed of estuaries or tidal deltas millions of years ago.”
Skookumchuck’s dramatic tides hint at a much larger, fantastically complex process that ripples through the solar system. The effect of global tidal friction actually acts as a brake on the earth’s rotation, making each day 1/50,000 second longer. And as tidal braking slows the earth’s rotation, energy is transferred by angular momentum to the moon, speeding it up and pushing it away at a rate of about an inch and a half per year, or ten feet in a human lifetime.
These numbers may seem negligibly small. And measured day to day they are. But over geological time, they add up. Scientists believe that 400 million years ago the earth’s day was only twenty-one hours long and the moon was about ten thousand miles closer. To earth’s earliest inhabitants, the moon would have loomed 20 percent larger than today. Tides, too, would have been larger and more frequent.
Richard Brathwaite didn’t know that time was slowing down when he wrote “Time and tide stayeth for no man.” In his day — the early seventeenth century — it was commonly understood that time and tide were reliably constant. Neither could be changed or stopped. The twenty-four-hour day was the time it took the earth to make one full rotation, the month was the time it took the moon to orbit the earth, and the year was the time it took the earth to circle the sun. By the late seventeenth century, Isaac Newton had described a well-behaved universe whereby time and motion followed predictable rules. And while Newton would have understood the concept of friction elsewhere, it was not factored into his tide model.
But less than a decade after the 1687 publication of Newton’s Principia Mathematica, Edmund Halley noticed that something was awry in the heavens. An accomplished scholar who served for twenty-two years as Britain’s astronomer royal, Halley discovered that ancient eclipses had occurred earlier than existing calculations indicated they should have. If the earth rotated at a uniform speed over geological history, Halley reasoned, it would follow that eclipses, which are the result of highly specific and predictable alignments of the sun and moon, could be traced to an exact place and time. But when he did the math, it turned out not to be so. Was time changing?
Luckily for Halley, eclipses had been observed and recorded for at least several thousand years. The Babylonians, Chinese, and Arabs kept the earliest surviving records, many of them etched by priests on clay tablets or parchment. In these, Halley found discrepancies. An eclipse recorded in Syria in 910 ce, for example, occurred half an hour earlier than it should have.
Perplexed, and under the influence of his friend Newton’s orderly theories, Halley pursued the subject only so far as to request eclipse observations from other travelers. It never occurred to him that his discovery was evidence that the earth’s rotation was slowing down and the length of a day was growing longer, much less that tides were the cause. If this were true—as we now know it is—then time itself could no longer be seen as constant and predictable.
Progress in understanding Halley’s discovery came slowly and in pieces over the following 250 years. For most normal people, including those of us living today, the concept of variable time is hard to digest. Physicists and astronomers are better equipped to understand it, but the rest of us don’t think of time as dependent on how much friction there is in the daily movement of tides across our watery planet. Yet it is.
In 1754, a half-century after Halley, the Berlin Royal Academy of Sciences put forth the following questions: “Has the earth undergone any alteration since the first period of origin? If it has, what would be the cause, and what can make us certain of it?” A response came from a twenty-four-year-old philosopher of metaphysics, Immanuel Kant, who suggested that the earth’s rotation had indeed fluctuated over time. Tides, he predicted, were the cause.
Another century passed before the thread was picked up again, this time by physicist and astronomer George Darwin (Charles Darwin’s third son), who was a scholar of lunar history. In his 1889 book The Tides and Kindred Phenomena, he hypothesized that roughly a billion years ago the earth had no moon. The day was only a few hours long, and hundred-foot tides sloshed so violently that they ripped the moon from the bottom of the Pacific and heaved it into space, only to be captured by the earth’s gravity while still close—a quarter the distance it is today. According to Darwin, the earth’s day was lengthening and the moon drifting away ever since, due to tidal friction and, to a lesser degree, friction in the body of the earth.
Darwin’s theory of the moon’s origin, known as fission, was disproved in the early twentieth century, but his hypothesis that tides were the cause of the changing orbital speeds and distances of the earth and moon is today accepted fact. Darwin understood this, but he couldn’t prove it.
Proof—or the hope of it—came a half-century later when Cornell paleontologist John Wells discovered a curious ring pattern in reef corals. “Corals,” he wrote in 1963, “lay down daily rings made of calcium carbonate much like a tree lays down rings during the fast- and slow-growing seasons of the year. Some coral species lay down both daily and yearly rings.” Thus, just as we can count tree rings to determine their age, we can count certain coral rings to determine the days per year.
Coral, composed of colonies of small animals, have lived on earth for a very long time, perhaps more than 500 million years. Each generation builds atop the one that just died, so over time a mountain is formed, with the youngest coral on top and the oldest at the bottom. The youngest—the ones living today—have ring counts of between 360 and 375, which roughly corresponds with the number of days in our current year. Coral that lived 600 million years ago, Wells found, have rings corresponding to a twenty-one-hour day.
Wells’s findings, admittedly rough, appeared in the journal Nature in 1963, inspiring a search for long-term records in other living sea fossils, such as scallops, mussels, clams, and algae.
Meanwhile, another source of proof appeared, this one from a reflector left on the moon during the 1969 Apollo landing. The device, not much larger than one of Buzz Aldrin’s nearby footprints, was designed to reflect a laser beam transmitted from earth. The beam’s travel time—almost the speed of light—could be converted to distance with accuracy within an inch. Over the last forty-seven years, the data has confirmed that the moon is receding at a rate of an inch and a half per year. It was a tremendous step forward in the effort to understand the earth-moon history, but forty-seven years is very short in geological time—not even a blink. What happened in the 4.5 billion years before that? Has the moon’s retreat been consistent since the beginning?
These questions are still largely unanswered, but research inspired by Wells continues. In the 1970s physicist Stephen Pompeii and geophysicist Peter Kahn thought they had found an animal that fit the criteria of being both very old and tuned to cosmic rhythms. The chambered nautilus, a relative of the squid and octopus, lives deep in the sea by day and swims to the surface by night. Not much is known about these creatures except that they’ve been around for about 500 million years.
“As nautilus grows,” wrote Pompeii and Kahn, “it incorporates two kinds of rhythmically repeating structures. One is the enlargement of the body chamber . . . and the other is septation, by which the parts of the shell abandoned by the animal are partitioned off into chambers.” Chambers are formed monthly, each sealed off with a wall as the animal spirals outward. Daily growth rings are laid down within each chamber. When Kahn and Pompeii counted these lines in nautiloids ranging in age from 25 to 420 million years, they found general agreement with Wells’s work.
Evidence of the earth’s rotational history has also come from the tide itself. Geologists have long known about tidal rhythmites, which are layers of soft stone formed on the seabed of estuaries or tidal deltas millions of years ago. These were once highly protected areas that allowed the tide to come and go with little disturbance from wind or waves. Consequently, each tide left a layer of sediment that varied in thickness, depending on the tide’s size and the current’s speed. Spring tides with faster currents, for example, left thin layers, while neap tides with slower currents left thick layers. These layers can be read in a laboratory to determine length of day, variations in tide range, and, by extrapolation, the moon’s distance.
In the 1990s very old rhythmites were discovered in Australia, France, the Netherlands, and even Utah, Alabama, and Indiana. A 620-million-year-old Australian rhythmite, when sliced in the lab, showed a day length of twenty-two hours, which correlated to an earth-moon distance of twelve thousand miles less than today’s. Some scientists are critical of this kind of research, particularly the coral and nautilus studies, arguing that ring counts are not reliably attributable to days, months, or years, or that data are biased toward desired results. But even if there’s not complete consistency in the ring counts, there’s enough consistency to make reasonable inferences. So far, it’s our only view into the ancient rotational history of the earth and moon. What rhythmites, corals, nautiluses, and even newly discovered eclipse records tell us is that, while the day is surely slowing and the moon is indeed drifting away, neither is doing so at a constant pace. Numerous factors account for this. Over geological time, tidal friction varies as continents drift or as periods of glaciation come and go (exposing more or less shallow water). Short-period events—earthquakes, volcanic eruptions, high winds over mountaintops (more friction)—can also jostle the earth’s rotation.
It seems our solar system is so tightly interwoven that not even the smallest dip or yaw goes unnoticed. In the end, what we’ve learned since Halley is that time is not constant or reliable. The earth’s day never was and never will be exactly twenty-four hours.
And tides are the reason.